Exploring the correlation analysis of immune microenvironment, mutation burden and prognosis of papillary thyroid carcinoma based on Estimate algorithm
Introduction
Papillary thyroid cancer is the most common endocrine malignancy worldwide (1). Over the past thirty years, the incidence rate of thyroid cancer has steadily increased (2), which may be related to improvements in diagnostic technology or environmental pollution (3). Papillary thyroid cancer is a well-differentiated thyroid cancer. Well-differentiated thyroid cancer maintains the typical differentiation characteristics of thyroid tissue, such as capturing iodine, synthesizing thyroglobulin, and responding to thyroid-stimulating hormone. These phenotypic characteristics gradually disappear when cancer cells change from well differentiated to poorly differentiated and undifferentiated cancer (4). Ninety percent of well-differentiated thyroid cancers have a relatively good prognosis. However, about 10% of patients still die due to the disease (5). More patients will face tumor recurrence. These differences may be due to tumor heterogeneity. Changes in the tumor microenvironment may lead to certain genetic and/or epigenetic changes in tumor cells, forming different subpopulations and acquiring heterogeneity (5). Some subgroups are more aggressive and less sensitive to conventional treatments (5).
Gene mutation and thyroid carcinogenesis and progression are closely related. The accumulation of gene mutations leads to changes in the phenotype of the cells themselves, malignant cells continue to proliferate, and finally form malignant tumors (1,6). Gene mutations may lead to changes in the immune microenvironment and enhance the ability of tumor cells to escape from immunity. Gene mutation and tumor immune escape is a dynamic process. However, as genetic mutations continue to accumulate, tumors are eventually able to evade the immune response entirely.
In recent years, with the development of gene databases and biotechnology, new gene targets are constantly being discovered. Thyroid cancer is caused by the involvement of gene mutations in many different ways, including rearranged during transfection proto-oncogene (RET), rat sarcoma (RAS) gene family, B-Raf proto-oncogene (BRAF), etc. previously discovered (1). Changes in the immune microenvironment have a significant impact on the biological behavior of tumor cells (7,8), which is one of the important features of tumor heterogeneity. Immunotherapy, which has been widely recognized as a treatment for solid tumors, is a good alternative treatment for papillary thyroid carcinoma with poor response to routine treatment (7). Gene mutation may be one of the driving forces for changes in the immune microenvironment (6). In thyroid cancer, the correlation between genetic mutations and the immune microenvironment is unclear. Both genetic mutations and the immune microenvironment have a significant impact on the biological behavior of thyroid cancer and determine the clinical phenotype. Exploring the relationship between gene mutations and the immune microenvironment might help to clarify the immune escape and chemotaxis mechanisms of tumor cells and better explain the biological heterogeneity of papillary thyroid carcinoma, thereby improving the diagnosis and treatment of this disease.
In this study, the Estimate algorithm was used to calculate the infiltration content of immune cells and stromal cells in thyroid cancer, to characterize the immune microenvironment, and to use the tumor mutation load to characterize the genomic mutation panorama of thyroid cancer. We macroscopically analyzed the correlation between the degree of immune cell infiltration and genomic alterations in papillary thyroid cancer, and their value in evaluating the prognosis of thyroid cancer patients. Our study may discover potential driver genes for thyroid cancer immune cell infiltration and provide a basis for thyroid cancer typing. We present the following article in accordance with the REMARK reporting checklist (available at https://gs.amegroups.com/article/view/10.21037/gs-22-211/rc).
Methods
Data download
The whole transcriptome sequencing data set, mutation data set, and clinical data set of papillary thyroid carcinoma were downloaded from The Cancer Genome Atlas (TCGA) database, including 58 normal thyroid tissue samples and 509 papillary thyroid carcinoma tissue samples. The whole transcriptome sequencing data set was sorted into a gene expression matrix of normal thyroid and tumor samples, and the matrix was normalized. Only 487 cases of papillary thyroid cancer were downloaded from the TCGA database. The mutation data set was sorted into a papillary thyroid carcinoma gene mutation matrix. Clinical information such as patient age, gender, pathological stage, and progression-free survival time were extracted from the clinical data set and incorporated into the data matrix. The study was conducted in accordance with the Declaration of Helsinki (as revised in 2013).
Calculation of TMB, immune score, and stromal score
The downloaded mutation data were visualized using R software and maftools package, and the tumor mutational burden (TMB) of thyroid cancer samples was calculated. The formula for calculating the TMB of the papillary thyroid carcinoma samples was calculated as follows: sample TMB = number of gene mutations/size of exon region. The expression level of TMB was extracted by PERL (https://www.perl.org/get.html). The stromal scores and immune scores of the papillary thyroid carcinoma samples were calculated using the “Estimate” package of R software (The R Foundation for Statistical Computing, Vienna, Austria). The Estimate method finally determined the matrix signal gene set genes and immune signal genes, and based on the expression levels of these two gene sets, the ssGSEA algorithm was used to finally determine the Stromal score and the Immune score.
Subsistence analysis
Prognostic analysis was performed by combining TMB and patient progression-free survival time. The patients were divided into high TMB group and low TMB group based on the optimal cutoff value. Progression-free survival analysis was performed using Kaplan-Meier and Log-rank tests. In the same way, the relationship between stromal score, immune score and prognosis of patients with thyroid cancer was analyzed. TMB, immune score, stromal score combined with clinical information of patients, including patient age, gender, case stage, etc., were used to screen independent risk factors of progression-free survival in patients with thyroid cancer using univariate COX analysis and multivariate COX analysis, with P<0.05 as the screening standard.
Statistical analysis
The data used in this study were statistically analyzed using R software and related R packages. Based on comparing the differences between tumor and normal samples, regardless of the overall distribution, a nonparametric test was used. Rank-sum test was used to analyze immune and stromal score differences between normal and tumor tissues in papillary thyroid carcinoma. Pearson’s test was used to analyze the correlation between the immune score, stromal score, and TMB. Two-sided P<0.05 was considered statistically significant.
Results
Stromal score difference
The mean value of stromal score of the 509 cases of papillary thyroid carcinoma was lower than that of the 58 cases of normal thyroid tissue, and the difference was statistically significant (P=0.0082; Figure 1).
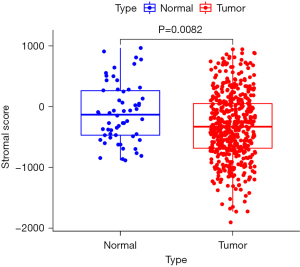
Immune score difference
The immune score of the 509 cases of papillary thyroid carcinoma was lower than that of the 58 cases of normal thyroid tissue, and the difference was statistically significant (P<2.22e-16; Figure 2).
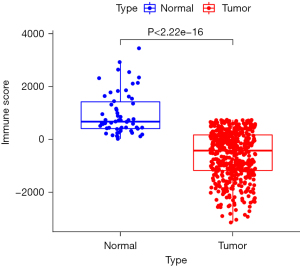
Stromal score survival analysis
The best cutoff value for the papillary thyroid carcinoma tissue stromal score was 243. The samples were divided into a high stromal score group (score >243, n=50) and a low stromal score group (score <243, n=448). There was no significant difference in progression-free survival between the high matrix and low stromal scores groups (P=0.075; Figure 3).
Immune score survival analysis
The best cutoff value for the papillary thyroid carcinoma tissue immune score was 470. The samples were divided into a high immune score group (score >470, n=57) and a low immune score group (score <470, n=441). The progression-free survival time of the high immune score group was better than that of the low immune score group, and the difference was statistically significant (P=0.029; Figure 4).
TMB survival analysis
The optimal cutoff value for the papillary thyroid carcinoma TMB was 0.42. The samples were divided into a high TMB group (score >0.42, n=55) and a low TMB group (score <0.42, n=428). The progression-free survival time of the low TMB group was better than that of the high TMB group (P<0.001; Figure 5).
Immune score combined with TMB survival analysis
The immune scores and TMB scores were combined, and the samples were divided into 4 groups: high immune score, high TMB; high immune score, low TMB; low immune score, high TMB; and low immune score, low TMB. There were significant differences in progression-free survival among the 4 groups. The prognosis of the high immune score, low TMB group was the best (P=0.003; Figure 6).
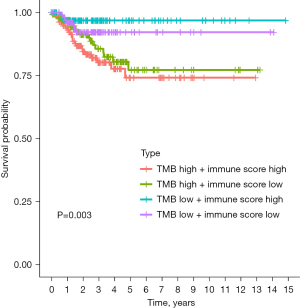
Univariate Cox analysis of progression-free survival
Univariate Cox analysis showed that age, pathological stage, and TMB were risk factors of progression-free survival [hazard ration (HR) >1, P<0.05], and that the immune score was a protective factor of progression-free survival (HR <1, P<0.05; Table 1).
Table 1
Factor | HR | 95% CI | P value | |
---|---|---|---|---|
Upper limit | Lower limit | |||
Age | 2.64 | 1.87 | 3.14 | 0.003 |
Sex | 0.82 | 0.34 | 1.06 | 0.214 |
Pathological stage | 1.21 | 1.03 | 2.14 | 0.037 |
Immune score | 0.85 | 0.36 | 0.89 | 0.003 |
Stromal score | 1.09 | 0.96 | 1.47 | 0.081 |
Sudden load | 1.71 | 1.45 | 2.51 | 0.004 |
HR, hazard ratio; CI, confidence interval.
Multivariate Cox analysis of progression-free survival
Multivariate Cox analysis showed that age and TMB were independent risk factors for progression-free survival (HR >1, P<0.05), and that the immune score was an independent protective factor for progression-free survival (HR <1, P<0.05; Table 2).
Table 2
Factor | HR | 95% CI | P value | |
---|---|---|---|---|
Upper limit | Lower limit | |||
Age | 2.04 | 1.51 | 2.89 | 0.008 |
Sex | 0.76 | 0.48 | 1.37 | 0.466 |
Pathological stage | 1.03 | 0.88 | 2.12 | 0.064 |
Immune score | 0.45 | 0.12 | 1.03 | 0.017 |
Stromal score | 1.19 | 0.83 | 1.63 | 0.122 |
Sudden load | 1.37 | 1.21 | 2.43 | 0.026 |
HR, hazard ratio; CI, confidence interval.
Correlation analysis between stromal score, immune score, and TMB
Correlation analysis showed that the stromal score and the immune score of papillary thyroid carcinoma were both negatively correlated with TMB (r=−0.26, P=0.031 and r=−0.41, P=0.028, respectively; Table 3).
Table 3
Correlation | r | 95% CI | P value | |
---|---|---|---|---|
Upper limit | Lower limit | |||
Immune score | −0.26 | −0.56 | −0.13 | 0.031 |
Stromal score | −0.41 | −0.73 | −0.20 | 0.028 |
TMB, tumor mutational burden; CI, confidence interval.
Discussion
This study used the whole transcriptome sequencing data of papillary thyroid carcinoma in the TCGA database to calculate the immune and stromal scores of the tumor samples. Immune and stromal scores indicate the abundance of immune and stromal cells in tumor tissue samples. The higher the immune and stromal scores, the higher the abundance of immune and stromal cells. In contrast, the lower the abundance of tumor cells, the lower the purity of the tumor. In our study, the immune and stromal scores in the normal tissue samples were significantly higher than those in the papillary thyroid carcinoma tissue samples. Our results showed that the immune score of the papillary thyroid carcinoma samples was associated with progression-free survival. Patients with a high immune score had a longer progression-free survival time than patients with a low immune score. However, the same correlation did not occur with the stromal score. These results may indicate that stromal cells have a weaker effect on the tumor than immune cells, particularly with respect to tumor inhibition.
In papillary thyroid carcinoma, the higher the abundance of immune cells, the better the prognosis of patients. Research has confirmed the inhibitory effect of immune cells on papillary thyroid carcinoma. Several studies have analyzed papillary thyroid carcinoma samples in TCGA using the CIBERSORT and TIMER deconvolution analysis tools and have confirmed that immune cell infiltration is related to histological type, mutation type, tumor pathological T stage, and lymph node N stage (9,10). Other studies have co-cultured T cells with papillary thyroid carcinoma cells to investigate the antitumor effect of T cells (10,11). These studies found that the biological behavior of papillary thyroid carcinoma cells changed significantly after co-culture, and that their invasion and migration abilities decreased. Tumor-associated macrophages are the most representative immune cell in the papillary thyroid carcinoma tumor microenvironment, and tumor-associated macrophage infiltration is related to the clinicopathological features of thyroid tumors. A study by Jung et al. showed that a high density of tumor-associated macrophages in the tumor immune microenvironment of thyroid cancer is associated with lower survival in both papillary thyroid carcinoma and anaplastic thyroid carcinoma (12). Another study found that an abundance of tumor-associated macrophages is positively correlated with lymph node metastasis and reduced survival in papillary thyroid carcinoma (13). In addition, tumor-associated macrophages are associated with capsular invasion and extrathyroidal extension (14). As an essential characteristic of papillary thyroid carcinoma, the tumor microenvironment may be a critical factor in determining the prognosis and treatment sensitivity of patients with papillary thyroid carcinoma.
This study confirmed that patients with papillary thyroid carcinoma with a high TMB had a poor prognosis. Thyroid tumors contain many mutations or fusion genes (15), which are related to the occurrence and progression of tumors. BRAF-driven mutations lead to constitutive activation of the mitogen-activated protein kinase 3 (MAPK) pathway, causing loss of differentiation, tumor progression, and inhibition of apoptosis (16,17). Telomerase reverse transcriptase (TERT) promoter mutations and tumor protein p53 (TP53) mutations are considered to be late events in thyroid tumorigenesis (15,18,19). When TP53 is mutated, it triggers uncontrolled cell replication and tumor development. P53 activation indicates the last step in tumor progression. These studies indicate that a higher tumor TMB is correlated with a worse prognosis, which is consistent with our results.
In thyroid cancer, gene mutation may lead to changes in the immune microenvironment that enhance the immune escape ability of tumor cells (6,20,21). The tumor establishes a dynamic balance with the immune system. In the initial stage of the cancer, immune surveillance recognizes tumor cells through the accumulated mutant genes. The immune system cannot eradicate these tumor cells and can only control the growth and continuous remodeling of the tumor. In the final stage of the disease, the progressive accumulation of gene mutations and modifications in the immune microenvironment enable the tumor to completely escape the immune response (20). The present study analyzed the relationship between gene mutation and immune infiltration. In thyroid cancer, immune and stromal scores were negatively correlated with TMB. These results suggested that thyroid cancer gene mutation might reduce the chemotaxis of immune cells.
In conclusion, the immune and stromal scores of papillary thyroid carcinoma were negatively correlated with TMB. Thyroid cancer gene mutations inhibit immune cell infiltration and alter the thyroid cancer microenvironment. The immune score was an independent protective factor for progression-free survival in patients with papillary thyroid cancer, while TMB was an independent risk factor, both of which can be used for clinical prognosis assessment. These results suggest that combined analysis of the immunology and genomics of papillary thyroid carcinoma can identify potential prognostic markers and therapeutic targets and provide clues for studying tumor immune escape mechanisms.
Acknowledgments
Funding: This work was supported by the National Natural Science Foundation of China (No. 81300346).
Footnote
Reporting Checklist: The authors have completed the REMARK reporting checklist. Available at https://gs.amegroups.com/article/view/10.21037/gs-22-211/rc
Conflicts of Interest: All authors have completed the ICMJE uniform disclosure form (available at https://gs.amegroups.com/article/view/10.21037/gs-22-211/coif). The authors have no conflicts of interest to declare.
Ethical Statement: The authors are accountable for all aspects of the work in ensuring that questions related to the accuracy or integrity of any part of the work are appropriately investigated and resolved. The study was conducted in accordance with the Declaration of Helsinki (as revised in 2013).
Open Access Statement: This is an Open Access article distributed in accordance with the Creative Commons Attribution-NonCommercial-NoDerivs 4.0 International License (CC BY-NC-ND 4.0), which permits the non-commercial replication and distribution of the article with the strict proviso that no changes or edits are made and the original work is properly cited (including links to both the formal publication through the relevant DOI and the license). See: https://creativecommons.org/licenses/by-nc-nd/4.0/.
References
- Ioachimescu AG. Thyroid Cancer. Endocrinol Metab Clin North Am 2019;48:xv-xvi. [Crossref] [PubMed]
- Cabanillas ME, McFadden DG, Durante C. Thyroid cancer. Lancet 2016;388:2783-95. [Crossref] [PubMed]
- Takano T. Overdiagnosis of Juvenile Thyroid Cancer. Eur Thyroid J 2020;9:124-31. [Crossref] [PubMed]
- D'Amore A, Patrone R, Docimo L, et al. Thyroid Cancer: Toward Surgical Evolution. J Clin Med 2021;10:3582. [Crossref] [PubMed]
- Chmielik E, Rusinek D, Oczko-Wojciechowska M, et al. Heterogeneity of Thyroid Cancer. Pathobiology 2018;85:117-29. [Crossref] [PubMed]
- Eszlinger M, Khalil M, Gillmor AH, et al. Histology-based molecular profiling improves mutation detection for advanced thyroid cancer. Genes Chromosomes Cancer 2021;60:531-45. [Crossref] [PubMed]
- Iesato A, Nucera C. Tumor Microenvironment-Associated Pericyte Populations May Impact Therapeutic Response in Thyroid Cancer. Adv Exp Med Biol 2021;1329:253-69. [Crossref] [PubMed]
- Jarboe T, Tuli NY, Chakraborty S, et al. Inflammatory Components of the Thyroid Cancer Microenvironment: An Avenue for Identification of Novel Biomarkers. Adv Exp Med Biol 2021;1350:1-31. [Crossref] [PubMed]
- Sun J, Shi R, Zhang X, et al. Characterization of immune landscape in papillary thyroid cancer reveals distinct tumor immunogenicity and implications for immunotherapy. Oncoimmunology 2021;10:e1964189. [Crossref] [PubMed]
- Ferrari SM, Fallahi P, Galdiero MR, et al. Immune and Inflammatory Cells in Thyroid Cancer Microenvironment. Int J Mol Sci 2019;20:4413. [Crossref] [PubMed]
- Yang Z, Wei X, Pan Y, et al. A new risk factor indicator for papillary thyroid cancer based on immune infiltration. Cell Death Dis 2021;12:51. [Crossref] [PubMed]
- Jung KY, Cho SW, Kim YA, et al. Cancers with Higher Density of Tumor-Associated Macrophages Were Associated with Poor Survival Rates. J Pathol Transl Med 2015;49:318-24. [Crossref] [PubMed]
- Qing W, Fang WY, Ye L, et al. Density of tumor-associated macrophages correlates with lymph node metastasis in papillary thyroid carcinoma. Thyroid 2012;22:905-10. [Crossref] [PubMed]
- Ryder M, Ghossein RA, Ricarte-Filho JC, et al. Increased density of tumor-associated macrophages is associated with decreased survival in advanced thyroid cancer. Endocr Relat Cancer 2008;15:1069-74. [Crossref] [PubMed]
- Song YS, Lim JA, Park YJ. Mutation Profile of Well-Differentiated Thyroid Cancer in Asians. Endocrinol Metab (Seoul) 2015;30:252-62. [Crossref] [PubMed]
- Landa I, Ibrahimpasic T, Boucai L, et al. Genomic and transcriptomic hallmarks of poorly differentiated and anaplastic thyroid cancers. J Clin Invest 2016;126:1052-66. [Crossref] [PubMed]
- de Biase D, Cesari V, Visani M, et al. High-sensitivity BRAF mutation analysis: BRAF V600E is acquired early during tumor development but is heterogeneously distributed in a subset of papillary thyroid carcinomas. J Clin Endocrinol Metab 2014;99:E1530-8. [Crossref] [PubMed]
- Landa I, Ganly I, Chan TA, et al. Frequent somatic TERT promoter mutations in thyroid cancer: higher prevalence in advanced forms of the disease. J Clin Endocrinol Metab 2013;98:E1562-6. [Crossref] [PubMed]
- Tiedje V, Ting S, Herold T, et al. NGS based identification of mutational hotspots for targeted therapy in anaplastic thyroid carcinoma. Oncotarget 2017;8:42613-20. [Crossref] [PubMed]
- Menicali E, Guzzetti M, Morelli S, et al. Immune Landscape of Thyroid Cancers: New Insights. Front Endocrinol (Lausanne) 2020;11:637826. [Crossref] [PubMed]
- Rotondi M, Coperchini F, Latrofa F, et al. Role of Chemokines in Thyroid Cancer Microenvironment: Is CXCL8 the Main Player? Front Endocrinol (Lausanne) 2018;9:314. [Crossref] [PubMed]
(English Language Editor: C. Gourlay)