Effect of intravenous lidocaine infusion on perioperative cellular immunity and the quality of postoperative recovery in breast cancer patients: a randomized controlled trial
Introduction
Breast cancer is a major global health problem, with more than 2 million new cases worldwide. It is both the most common malignancy and the leading cause of cancer death in women (1). Although surgical resection under general anesthesia is still the main treatment for breast malignancies, the perioperative immunosuppression induced by surgery and anesthesia can cause the immunologic escape of tumor cells and tumor metastasis (2). Studies have shown that local anesthetics can affect the apoptosis, proliferation, metastasis, and oncogene DNA demethylation of certain tumor cells to regulate the immune system (3-6). However, there are few clinical studies on the effects of local anesthetics on the perioperative cellular immunologic function of patients with malignancies (7,8). Despite surgical treatment, 6% of patients with local tumors and 22% of patients with lymphatic metastasis will be confronted with recurrence within 5 years (9). Accordingly, there is growing concern that the perioperative period contributes directly (i.e., through oncologic manipulation) or indirectly to the spread of tumors. On the one hand, tumor surgery is crucial in the treatment of solid tumors, as final resection can achieve a complete cure, while on the other hand, it may increase the risk of microproliferation and further clinical metastasis. The development of clinical metastasis also depends on the balance between the growth ability of cancer cells at the site of metastasis and the patient’s ability to fight the tumor (i.e., the anti-tumor immunity of the host) (10). Therefore, the recovery of the patient’s immunologic function after surgery is also crucial for tumor prognosis. A variety of anesthetic drugs are required during the perioperative period, and current studies have shown that opioids and inhalation anesthetics have immunosuppressive effects, weakening the resistance of tumor patients to invasion by residual tumor cells during the perioperative period, which may be detrimental to tumor prognosis. Local anesthetics and propofol can directly affect the growth and invasion of tumor cells, which may help to reduce tumor recurrence and improve patient prognosis (7). Among them, the effects of regional block anesthesia and local anesthetics on postoperative metastatic recurrence in tumor patients are of the most concern, which has promoted more studies of their potential mechanisms.
Local anesthetics can be applied alone or in combination with general anesthetics. The application of local anesthetics keeps down the demand for volatile anesthetics and opioids while alleviating the neuroendocrine stress response and perioperative immunosuppression. The results of Hu et al. found that ultrasound-guided erect spinal plane (ESP) block had little effect on immune function in patients undergoing radical mastectomy and promoted postoperative recovery (11). In recent years, intravenous lidocaine has attracted great interest from clinicians as part of the perioperative anesthetic regimen because of its analgesic, anti-hyperalgesia, and anti-inflammatory effects and its resultant shortened hospital stay. Lidocaine may also have the potential to reduce the risk of postoperative recurrence or progression in patients undergoing cancer surgery (12,13). A large number of in vitro experiments and some animal experimental evidence currently suggest that lidocaine may inhibit tumor recurrence, but there is still a lack of robust clinical studies demonstrating its benefit in patients undergoing tumor surgery (14). Wang et al. found that continuous intraoperative lidocaine infusion was protective of cell-mediated immune function in patients with cervical cancer undergoing radical hysterectomy (15). In the randomized, double-blind, placebo-controlled trial by Hou et al., they randomly assigned 60 patients with early-stage non-small cell lung cancer (NSCLC) undergoing video-assisted thoracic surgery (VATS) to either a lidocaine group (n=30; intravenous push lidocaine 1.0 mg/kg, 1.0 mg/kg/h until surgery end of surgery) or saline control group (n=30). The primary outcome was serum IL-17 levels at 24 hours postoperatively. The results showed that intravenous lidocaine was associated with a decrease in serum IL-17 and cortisol after surgery in patients with early NSCLC (16). However, their studies all ignored the immune status of the preoperative organism and the effects of different degrees of surgical stress on the immune system. There are many factors that affect the immune function of the body during the perioperative period. In this study, we recorded preoperative leukocyte and lymphocyte counts, excluded patients who had received preoperative radiotherapy, and patients who had intraoperative blood transfusions, and all patients were operated on by the same surgical team. This study aims to investigate the effect of lidocaine on postoperative cellular immunity in patients with breast cancer, in order to provide a promising approach for improving postoperative prognosis. We present the following article in accordance with the CONSORT reporting checklist (available at https://gs.amegroups.com/article/view/10.21037/gs-22-134/rc).
Methods
This study is a prospective single-center, double-blind, randomized controlled trial conducted in the Department of Anesthesiology of the Affiliated Hospital of Xuzhou Medical University. The study protocol was approved by the Ethics Committee of the Affiliated Hospital of Xuzhou Medical University (No. XYFY2019-JS017-02), with all patients or their family members signing informed consent. The study was conducted in accordance with the Declaration of Helsinki (as revised in 2013).
Participants
A total of 62 female patients undergoing an elective modified radical mastectomy in our hospital from December 2019 to April 2021 were selected and divided into 2 groups according to a computer-generated random digit table in a 1:1 ratio, namely, the control group (Group S, n=31) and the lidocaine group (Group L, n=31).
- Inclusion criteria: 18–85 years old; female patients; proposed to undergo elective unilateral radical mastectomy; confirmed diagnosis of primary invasive breast cancer (TNM staging: T1-3, N0-2, M0) on frozen pathological sections; agreed to participate in this study and signed the informed consent.
- Exclusion criteria: allergic to local anesthetics such as lidocaine; history of chemotherapy or radiotherapy and patients undergoing secondary surgery (recurrence or reconstruction); metastatic breast cancer and inflammatory breast cancer; BMI >30 kg/m2; endocrine system diseases, autoimmune diseases, immunodeficiency diseases, other active cancers and recent (<1 month) history of infection, or patients with American Society of Anesthesiologists (ASA) status IV-V, leukemia, or the long-term use of immunosuppressive drugs; unable to cooperate with the researcher for any reason; hearing or a visual impairment, speech comprehension impairment, mental illness; pregnant or breastfeeding; comorbid central neuropathy, hepatic or renal dysfunction and hypotension (systolic blood pressure ≤90 mmHg); those who have participated in other studies.
- Rejection criteria: patients with perioperative transfusion of blood products; patients with intraoperative application of local anesthesia (epidural anesthesia, nerve block, local incision infiltration) or intraoperative application of chemotherapeutic drugs; those who voluntarily withdrew from the trial; emergency unblinding cases; those who did not obey the arrangement or agreement of the trial.
- Trial termination: breaking of blindness; ethics committee termination or suspension of the trial; emergency envelope unblinding rate exceeding 20%; mid-term finding of a significantly higher rate of complications or adverse events in the intervention group than in the control group.
Randomization, blinding, and allocation concealment
A simple randomization method was used to randomly group the subjects, and the subjects were randomly grouped at a ratio of 1:1 using random numbers generated by a computer probabilistic system. The preparation of the intravenous infusion drug for patients in both groups was performed independently by an anesthesia nurse who did not participate in this study. The syringes containing the preparation were labeled with the patient’s name, date of preparation, and route of administration, hence the blinding staff could not determine whether the preparation belonged to the control group or test group based on the appearance of the preparation. Emergency envelopes were prepared at the same time as drug blinding, and an opaque emergency envelope was set up for each blind number, which contained the case medication number and drug name so that individual cases could be unblinded and resuscitated in case of emergency. After the unblinding, the corresponding case was treated as a shedding case. The emergency envelope was sent to the researcher along with the already-blinded drugs. This was a double-blind trial, and all the patients and their families, anesthesiologists, surgeons, study recorders, and evaluators were unaware of the grouping and the composition of the drugs dispensed. Additionally, neither the blood specimen monitoring personnel nor the intraoperative pathology staff had any knowledge of the trial groupings. A supervisor dedicated to coordinating and overseeing the entire research supervised the implementation of the blinding method, ensuring the safety of the subjects and the reliability of the results. This supervisor was responsible for distributing emergency envelopes, maintaining the blind codes, and unblinding at the end of the trial.
Anesthesia and post-anesthesia management
All patients were routinely requested to undergo solid food fasting and liquid fasting before surgery. The data collector visited the patients 1 day before surgery, explained the meaning and use of the visual analogue scale (VAS) to the patients. The scale consists mainly of a 10-cm line, with one end of the line indicating “no pain at all” and the other end indicating “the most severe pain imaginable”, “pain to the extreme” etc. Patients are asked to mark the appropriate place on the line (with a dot or an “×”, etc.) to represent the intensity of the pain they are experiencing at that time (17). After admission, all patients were monitored for non-invasive blood pressure (NIBP), electrocardiogram (ECG), and pulse oxygen saturation (SpO2). Upper limb venous access was opened and an intravenous infusion of 8 mL/kg compound electrolytes was administered.
The bispectral index (BIS) was monitored by a brain state index monitor. General anesthesia was induced by 0.05 mg/kg intravenous midazolam, 0.05 µg/kg sufentanil, 0.9 mg/kg rocuronium, and 0.4 mg/kg etomidate, and the laryngeal mask was inserted when the patient’s neck muscles were relaxed and the BIS value decreased to 40–60. Respiratory parameters were set as follows: the total flow of fresh gas was adjusted to 2 L/min (FiO2 0.5), tidal volume to 6–8 mL/kg, and respiratory rate to 12–14 times/min. Intraoperative respiratory parameters were adjusted according to the situation, maintaining End-expiratory carbon dioxide partial pressure (PETCO2) at 35–45 mmHg (1 mmHg = 0.133 kPa). All patients were given 4 mg intravenous tropisetron before skin excision to prevent postoperative nausea and vomiting.
General anesthesia was maintained with propofol (4–6 mg/kg/h) and remifentanil to maintain BIS values between 40 and 60. The infusion volume of remifentanil was adjusted as needed to maintain the mean arterial pressure (MAP) within ±20% of baseline values. Hypotension was defined as exceeding or decreasing 20% of the baseline MAP, or MAP <65 mmHg for more than 30 s.
When hypotension occurred, 20 µg phenylephrine was administered. The intraoperative heart rate (HR) was maintained at 60–100 beats/min. When bradycardia (HR <50 beats/min) was present, 0.5 mg atropine was administered. A convective warming blanket was used to maintain the patient’s intraoperative body temperature at 36–37 ℃. Anesthetics were stopped during skin suturing, then 0.04 mg/kg neostigmine, 0.02 mg/kg atropine, and 0.5 mg flumazenil were injected intravenously, and the patient was sent to the post-anesthesia care unit (PACU). When the patient was awake with spontaneous breathing, laryngeal reflex, and cough reflex restored, and the tidal volume and minute respiratory volume normalized, the laryngeal mask could be removed and oxygen was administered with a face mask at 3 L/min. After observation in the PACU for about 1 h, the patient could return to the ward. All patients were given standard postoperative analgesia. If the postoperative VAS score exceeded 4 points, 50 mg flurbiprofen axetil would be injected intravenously. If the score was still greater than 4 points, 0.1 mg fentanyl would be injected intravenously.
Intervention
In addition to the standard intraoperative management described above, a loading dose of 1.5 mg/kg of 2% lidocaine was pumped intravenously into the patients of Group L 10 min before induction of anesthesia, followed by induction of anesthesia using mask ventilation for oxygen denitrogenation. After the induction of anesthesia, this drug was continued at a pumping rate of 2.0 mg/kg/h using a micropump until the end of surgery. In Group S, a loading dose of normal saline with pumping was applied in an equal volume.
Outcomes and data collection
The primary outcome indicator was the change in natural killer (NK) cell levels in peripheral blood during the perioperative period. The secondary outcome indicators were as follows: changes in T lymphocyte subset levels in peripheral blood during the perioperative period; patients’ QoR-15 scores were recorded by questionnaire before and 24 h after the operation; intraoperative dosage of propofol and remifentanil; additional use of postoperative analgesics and the incidence of complications (postoperative nausea and vomiting, dizziness, and chest tightness). The safety indexes were the occurrence of intraoperative arrhythmias and lidocaine intoxication.
Blood specimens
A volume of 3 mL of venous blood from the contralateral elbow of the enrolled patients was collected 30 min before surgery (T0), after tumor resection (T1), immediately after surgery (T2), 24 h after surgery (T3), and 48 h after surgery (T4). Whole blood samples were anticoagulated with heparin, stored at room temperature, and sent for examination within 4 h.
Flow cytometry
For the preparation of phosphate buffered saline (PBS), 16.00 g NaCl, 0.40 g KCl, 2.88 g NaH2PO4, and 0.48 g K2HPO4 were weighed then dissolved in 1,800 mL of tri-distilled water. The pH was adjusted to 7.4 with HCl, then tri-distilled water was added to a constant volume of 2,000 mL. The solution was autoclaved and stored at room temperature. For the preparation of tris-NH4Cl (erythrocyte lysate), 7.47 g of NH4Cl (ammonium chloride) and 1.3 g of Tris (trihydroxymethyl aminomethane) were weighed and dissolved in 1,000 mL of water, then the solution was stored at 4 ℃.
A volume of 200 µL of venous blood was added into a flow tube (Nanjing Beyotime Biotechnology Co., Ltd.), then the appropriate amount of antibody was added [PerCP/Cyanine5.5 anti-human CD4; APC anti-human CD8a; FITC anti-human CD3; PE anti-human CD56 (NCAM); PE anti-human CD16; PE/Cyanine7 anti-human CD45; PE Mouse IgG2a, κ Isotype Ctrl; PE Mouse IgG1, κ isotype Ctrl; Biolegend, USA]. After mixing well and incubating in the dark for 20 min, 2 mL of erythrocyte lysate was added and mixed well to lyse the erythrocytes. Then, 2 mL of PBS was added and mixed well, followed by centrifugation at 2,000 r/min (Beckman Coulter, USA). After washing 3 times, 200 µL of PBS was finally added and mixed well. Samples were placed in the dark at 4 ℃ for experiments, and the results were recorded after detection by flow cytometry (FACS Calibur flow cytometer, Becton, Dickinson and Company, USA).
Sample size
Based on the results of the preliminary experiment (unpublished data, n=20), the sample size was estimated using repeated measurement data. To prove that the mean discrepancy of NK cell percentage was 2%, the standard deviation was 4.2%, and the autocorrelation coefficient between adjacent measurement points of the same tester was 0.3, a two-sided test was adopted, with a test efficacy of 0.05 and a 90% certainty. PASS 15.0 (NCSS, LLC, Kaysville, USA) was applied for calculation, the sample size was 30 cases per group, and an expulsion rate of 10% would be taken into consideration. Eventually, 68 cases of breast cancer patients were to be recruited for this study.
Statistical analysis
For continuous data, the normality test was carried out first, and if each group met the normality criteria and the variances between the 2 groups were equal, the t-test would be adopted for comparisons between groups; otherwise, a nonparametric Wilcoxon rank-sum test was considered. For grouped data, the chi-square test was used for unordered outcomes, while the nonparametric Wilcoxon rank-sum test was adopted for ordered data. In this study, due to the multiple repeated measurements, we used repeated measurement analysis of variance (ANOVA) for comparisons of continuous variables and variables of the compound normal distribution; otherwise, we used a generalized estimating equation for comparison. The groups were compared separately to determine statistical differences between groups and time points, and the interaction terms between groups and time points were analyzed to explore whether there were differences in the changing trend between groups. If the interaction terms were statistically significant, a simple effects analysis would be performed to analyze the trend of change over time for each group. All statistical analyses were performed using a two-sided test, with P<0.05 representing a statistically significant difference. All statistical analyses were conducted using SPSS 25.0 (SPSS, Inc., Chicago, IL, USA).
Results
Female patients undergoing elective modified radical mastectomy in our hospital from December 2019 to April 2021 were selected. There were 68 primary screening patients, among which 6 (9%) did not meet the inclusion criteria or met the exclusion criteria, and 2 refused to participate in the study. At first, a total of 62 (91%) patients were enrolled. One patient in Group S voluntarily withdrew from the trial, while 1 patient’s blood sample in Group L did not meet the requirements, and they were lost to follow up. Finally, 60 (88%) patients were statistically analyzed, with 30 patients in each group (Figure 1). The general information of the patients in both groups was well balanced (Table 1).
Table 1
Variables | Group L | Group S | P value |
---|---|---|---|
Age (years) | 50.02±9.47 | 50.67±8.54 | 0.842 |
BMI (kg/m2) | 24.53±2.25 | 24.44±2.29 | 0.875 |
ASA classification | 0.756 | ||
I | 23 (77%) | 24 (80%) | |
II | 7 (23%) | 6 (20%) | |
TNM stage | 0.829 | ||
I | 7 (23%) | 7 (23%) | |
II | 21 (70%) | 22 (73%) | |
III | 2 (7%) | 1 (4%) | |
Preoperative total lymphocyte count (×109/L) | 2.05±0.62 | 2.12±0.58 | 0.690 |
Preoperative white blood cell count (×109/L) | 6.09±1.20 | 5.94±1.11 | 0.616 |
Surgery types (%) | 0.595 | ||
Breast-conserving surgery | 10 (33%) | 12 (40%) | |
Unilateral modified radical mastectomy | 20 (67%) | 18 (60%) | |
Surgery time (min) | 147.03±63.02 | 143.27±55.86 | 0.807 |
Intraoperative fluid infusion volume (mL) | 1,322.27±365.12 | 1,301.80±364.14 | 0.829 |
Blood loss (mL) | 106.80±19.90 | 108.57±21.56 | 0.593 |
Data are presented as mean ± SD or n (%). BMI, body mass index; ASA, American Society of Anesthesiologists; TNM, Tumor Node Metastasis.
As shown in Figure 2, there was a significant difference in the percentage of NK cells between the 2 groups (F=7.675, P=0.008), and the percentage of NK cells in Group S was lower than that in Group L. The measurement time also had an impact (F=32.766, P<0.001). The percentage of perioperative NK cells in both groups began to decline at T1 and then showed a downward trend over time to the lowest level at T2. Then, they presented an upward trend at T3, but the upward trend of Group S during the T2-T3 period was not significant (the differential value was 0.54, 95% CI: –1.67 to 2.75, P=0.999). The upward trend during T3-T4 was greater (the differential value was 4.86, 95% CI: 2.44 to 7.27, P<0.001), but the percentage of perioperative NK cells during this period did not restore to the initial level. In Group L, the restoration of NK cells was obvious during T2-T3 (the differential value was 5.49, 95% CI: 3.28 to 7.70, P<0.001), and reached baseline level at T3 (the differential value was –1.94, 95% CI: –5.20 to 1.32, P=0.602), but the trend slowed down during T3–T4 (the differential value was 1.75, 95% CI: –0.65 to 4.17, P=0.324). Furthermore, there was an interaction effect between group and time (F=7.328, P<0.001). As measurement time increased, the decrease in the percentage of NK cells and the recovery trend differed between the 2 groups. After comparing the 2 groups, the percentage of NK cells of Group L was significantly higher than that of Group S at T3 (the differential value was 7.04, 95% CI: 4.57 to 9.51, P<0.001) and T4 (the differential value was 3.94, 95% CI: 0.91 to 6.97, P=0.012). At T1 and T2, though the percentage of NK cells in Group L was higher than that of Group S, the difference was not statistically significant (P>0.05).
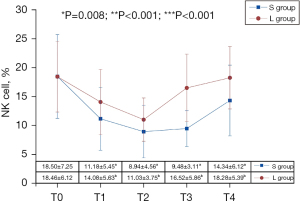
Different counts of perioperative CD3+ T cells (F=19.903, P<0.001), CD4+ T cells (F=4.515, P=0.038), and the CD4+/CD8+ value (F=4.400, P=0.040) between the 2 groups were statistically significant. The data in Group S were lower than that in Group L, and there was an interaction effect between group and time. The influence of lidocaine on the counts of CD3+ T cells, CD4+ T cells, and the CD4+/CD8+ value changed with time, whereas no significant difference was found between the 2 groups in terms of CD8+ T cells (F=1.936, P=0.169) and time and group interaction. The counts of CD3+ T, CD4+ T, and CD8+ T cells differed at different times (P<0.001). At T1, the counts of the CD3+ T, CD4+ T, and CD8+ T cells of both groups began to decline and decreased to the lowest level at T2 (P<0.05). At T3, they showed an upward recovery trend. In contrast, the CD4+/CD8+ value in Group S showed a declining trend at T1 and T2 then went up at T3 and T4, reaching the baseline level at T4 (P>0.05). However, the CD4+/CD8+ value in Group L showed an increasing trend at T1, T2, T3, and T4, with a faster increase at T1 and T2 (P<0.05) and a slower increase at T3 and T4 (P>0.05). The percentage of CD3+ T cells in Group S was significantly lower than that in Group L at T1, T2, T3, and T4 (P<0.05). The percentage of CD4+ T cells and the CD4+/CD8+ value in Group L were significantly higher than those in Group S at T2, T3, and T4 (P<0.05). However, at T1, though not significantly, the percentage of CD4+ T cells and the CD4+/CD8+ value in Group L were also higher than those in Group S, but there was no statistical significance (P>0.05). The difference in CD8+ T cells between the 2 groups was statistically significant at T5 (P<0.05), but not at other time points (P>0.05). Details are revealed in Figure 3.
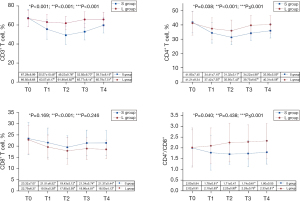
The preoperative QoR-15 scores were 133.50 (12.50) in group S and 136.00 (11.50) in group L. The preoperative QoR-15 score in the two groups was not statistically significant (P=0.134). The QoR-15 scores were significantly higher in the group L than in the group S on the 24 h postoperative. It was 128.50 (20.25) in group L and 117.50 (19.50) in group S (Table 2 and Figure 4). The difference was statistically significant (P=0.005).
Table 2
Variables | Group L (n=30) | Group S (n=30) | P value |
---|---|---|---|
Preoperative QoR-15 scores | 136.00 (11.50) | 133.50 (12.50) | 0.134 |
QoR-15 scores at 24 h after surgery | 128.50 (20.25) | 117.50 (19.50) | 0.005 |
Intraoperative propofol dosage (mg) | 908.16 (430.14) | 934.17 (531.72) | 0.836 |
Intraoperative lidocaine dosage (mg) | 257.50 (176.23) | 0 | 0.000 |
Intraoperative remifentanil dosage (μg) | 1,145.00 (531.25) | 1,385.69 (674.88) | 0.014 |
Frequency of using analgesics | 0 (1.00) | 1 (1.00) | 0.022 |
Postoperative nausea and vomiting | 2 (6.67%) | 5 (16.67%) | 0.436 |
Vertigo | 3 (10.00%) | 9 (30.00%) | 0.098 |
Chest distress | 6 (20.00%) | 9 (30.00%) | 0.226 |
Data are presented as M (IQR) or n (%). QoR-15, quality of recovery-15.
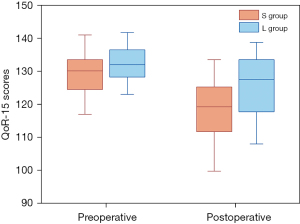
The intraoperative propofol dosage was 934.17 (531.72) mg in Group S and 908.16 (430.14) mg in Group L. The results showed no significant difference between the 2 groups (P=0.836). The intraoperative remifentanil dosage was 1,385.69 (674.88) µg in Group S and 1,145.00 (531.25) µg in Group L, presenting a significant difference (P=0.014). There was a significant difference between the 2 groups in the frequency of using analgesics (P=0.022). In Group S, 5 patients experienced postoperative nausea and vomiting, and 9 patients experienced vertigo and chest distress. In Group L, 2 patients had postoperative nausea and vomiting, 3 patients had vertigo, and chest distress occurred in 6 patients. The results showed no significant difference in the incidence of nausea and vomiting, vertigo, and chest distress between the 2 groups (P=0.436, P=0.098, P=0.226). More details are shown in Table 2. No perioperative adverse events such as cardiac arrhythmias and lidocaine toxicity occurred in either group.
Discussion
Surgical stress can exacerbate malignancy-induced immunosuppression, which may facilitate cancer recurrence (2). Perioperative risk factors for cancer recurrence include postoperative residual cancer cells, cancer-free margin, cancer cell characteristics, and immune function (18). The management of the tumor during surgery may boost metastasis. Other factors associated with tumor recurrence are anesthetic drugs, anesthetic techniques, acute pain, and the use of opioids. There is growing evidence that anesthetic techniques and anesthetic drugs may influence the long-term proliferation and recurrence of tumors (19). Perioperative use of anesthetic/analgesic techniques with protective effects against metastatic immune responses may slow tumor progression. The effects of different anesthetic drugs on immune cell function are different, and the anesthetic drugs that have the least effect on cellular immune function may optimize the prognosis of patients. Moreover, in in vitro and retrospective studies, the anti-metastatic effects and protective effects of lidocaine on immune function have received extensive attention. Therefore, this study examined the effects of perioperative intravenous infusion of lidocaine on cellular immune function and the quality of postoperative recovery.
Cellular immunity of NK cells and T lymphocyte subsets plays a crucial role in the body’s anti-tumor immune effect (20,21). NK cells are broad-spectrum killer cells that play an important role in inhibiting tumor proliferation and growth, and produce cytokines that regulate phagocytosis. They are also important immunomodulatory cells that regulate T lymphocytes, B lymphocytes, and bone marrow stem cells, among others, by releasing lymphokines (IFN, IL-2), preventing the spread of circulating tumor cells and inhibiting the viability of microscopic cancer foci that are freed during surgery (22). Therefore, the NK cell level reflects the immune status of the body to a certain extent. Some studies have shown that NK cell levels are higher in breast cancer patients before surgery than after surgery (23-25). The increase in the percentage of NK cells can improve the immune function of the body, giving it the ability to kill tumor cells. The results of our study showed that the percentage of NK cells, CD3+ cells, and CD4+ cells and the CD4+/CD8+ values were significantly higher in patients in Group L than in Group S patients at T1–T4, and the recovery time of immune cells was shorter in Group L than in Group S, indicating that perioperative intravenous pumping of lidocaine improved the cellular immune function in patients with primary invasive breast cancer after surgery. The overall immune function of the body was consistent with the level of CD3+ cells. By secreting a large number of cytokines that assist CD8+ cells in killing tumor cells, CD4+ cells played a vital role in anti-tumor immunity (26). When the postoperative cellular immune function was severely impaired, the counts of CD4+ and CD8+ cells changed between the 2 groups, disrupting the balance. Cellular immune dysfunction occurred with a decrease in immune cells, and several anesthetic drugs such as sufentanil, remifentanil, and sevoflurane caused suppression of cellular immune function, suggesting that cellular immune function was related to anesthesia (27). Therefore, the choice of the appropriate anesthetic drug was crucial for patients undergoing malignant tumor resection. Previous studies have demonstrated the benefits of propofol in anesthesia for patients with malignant tumors, and lidocaine has been shown to have antitumor effects (28,29). In this study, both methods of anesthesia had a suppressive effect on cellular immune function in patients with primary invasive breast cancer after surgery, but the results showed that continuous intravenous pumping of lidocaine had a less suppressive effect on NK cell and T cell immune function. Therefore, perioperative intravenously pumped lidocaine may be a better option for patients with primary invasive breast cancer.
Another important finding was that the quality of postoperative recovery was significantly higher in Group L than in Group S. In addition, the study also found a lower incidence of adverse events in Group L than in Group S, suggesting that perioperative intravenous pumping of lidocaine was effective and safe in primary invasive mastectomy. It is known that pain is an important risk factor for the recurrence of cancer metastasis (30). Based on these findings, this study provided further evidence that perioperative intravenous pumping of lidocaine helped improve the quality of patients’ postoperative recovery and reduce postoperative adverse reactions.
However, the small sample sizes and short follow-up periods of this study, which failed to record long-term prognostic indicators for patients, did not provide stronger evidence for our findings. Therefore, more evidence should be explored in further studies with larger sample sizes and longer follow-up periods. How to better preserve T lymphocyte and NK cell activity to reduce surgery-mediated neuroendocrine stress, maintain the homeostasis of the body’s microenvironment, and potentially enhance host immune function against tumor cells is the challenge for perianesthesia management for oncology surgery. An effective response to the suppression of the immune system by anesthetic drugs is a guarantee of a balanced perioperative microenvironment and provides the best prognosis for the patient. Maximizing the protection of the microenvironment and immune function in oncology surgery patients and optimizing patient prognosis are some of the directions and tasks for future studies in the field.
Conclusions
To sum up, continuous intravenous infusion of lidocaine promoted postoperative cellular immune function, reduced the amount of intraoperative remifentanil administered, improved the quality of patients’ postoperative recovery, and mitigated postoperative pain and adverse reactions. Therefore, it can be used for immune function protection in patients with primary invasive breast cancer with a better therapeutic effect, thereby improving the quality of the patient’s recovery.
Acknowledgments
The authors appreciate the support of the Thyroid and Breast surgeons and nursing teams of the Affiliated Hospital of Xuzhou Medical University.
Funding: None.
Footnote
Reporting Checklist: The authors have completed the CONSORT reporting checklist. Available at https://gs.amegroups.com/article/view/10.21037/gs-22-134/rc
Data Sharing Statement: Available at https://gs.amegroups.com/article/view/10.21037/gs-22-134/dss
Trial Protocol: Available at https://gs.amegroups.com/article/view/10.21037/gs-22-134/tp
Conflicts of Interest: All authors have completed the ICMJE uniform disclosure form (available at https://gs.amegroups.com/article/view/10.21037/gs-22-134/coif). The authors have no conflicts of interest to declare.
Ethical Statement: The authors are accountable for all aspects of the work in ensuring that questions related to the accuracy or integrity of any part of the work are appropriately investigated and resolved. The study was conducted in accordance with the Declaration of Helsinki (as revised in 2013). The study was approved by the Institutional Ethics Committee of the Affiliated Hospital of Xuzhou Medical University (No. XYFY2019-JS017-02), with all patients or their family members signing informed consent.
Open Access Statement: This is an Open Access article distributed in accordance with the Creative Commons Attribution-NonCommercial-NoDerivs 4.0 International License (CC BY-NC-ND 4.0), which permits the non-commercial replication and distribution of the article with the strict proviso that no changes or edits are made and the original work is properly cited (including links to both the formal publication through the relevant DOI and the license). See: https://creativecommons.org/licenses/by-nc-nd/4.0/.
References
- Siegel RL, Miller KD, Fuchs HE, et al. Cancer Statistics, 2021. CA Cancer J Clin 2021;71:7-33. [Crossref] [PubMed]
- Raigon-Ponferrada A, Recio MED, Guerrero-Orriach JL, et al. Breast Cancer and Anesthesia. Curr Pharm Des 2019;25:2998-3004. [Crossref] [PubMed]
- Liu H, Dilger JP, Lin J. Effects of local anesthetics on cancer cells. Pharmacol Ther 2020;212:107558. [Crossref] [PubMed]
- Zhu G, Zhang L, Dan J, et al. Differential effects and mechanisms of local anesthetics on esophageal carcinoma cell migration, growth, survival and chemosensitivity. BMC Anesthesiol 2020;20:126. [Crossref] [PubMed]
- Wu Chuang A, Kepp O, Kroemer G, et al. Direct Cytotoxic and Indirect, Immune-Mediated Effects of Local Anesthetics Against Cancer. Front Oncol 2022;11:821785. [Crossref] [PubMed]
- Xu P, Zhang S, Tan L, et al. Local Anesthetic Ropivacaine Exhibits Therapeutic Effects in Cancers. Front Oncol 2022;12:836882. [Crossref] [PubMed]
- Chamaraux-Tran TN, Mathelin C, Aprahamian M, et al. Antitumor Effects of Lidocaine on Human Breast Cancer Cells: An In Vitro and In Vivo Experimental Trial. Anticancer Res 2018;38:95-105. [PubMed]
- Galoș EV, Tat TF, Popa R, et al. Neutrophil extracellular trapping and angiogenesis biomarkers after intravenous or inhalation anaesthesia with or without intravenous lidocaine for breast cancer surgery: a prospective, randomised trial. Br J Anaesth 2020;125:712-21. [Crossref] [PubMed]
- Coughlin SS. Epidemiology of Breast Cancer in Women. Adv Exp Med Biol 2019;1152:9-29. [Crossref] [PubMed]
- Zamarron BF, Chen W. Dual roles of immune cells and their factors in cancer development and progression. Int J Biol Sci 2011;7:651-8. [Crossref] [PubMed]
- Hu Y, Li M, Li J, et al. Effects of ultrasound-guided erector spinae plane block on the immune function and postoperative recovery of patients undergoing radical mastectomy. Gland Surg 2021;10:2901-9. [Crossref] [PubMed]
- Dunn LK, Durieux ME. Perioperative Use of Intravenous Lidocaine. Anesthesiology 2017;126:729-37. [Crossref] [PubMed]
- Choi KW, Nam KH, Lee JR, et al. The Effects of Intravenous Lidocaine Infusions on the Quality of Recovery and Chronic Pain After Robotic Thyroidectomy: A Randomized, Double-Blinded, Controlled Study. World J Surg 2017;41:1305-12. [Crossref] [PubMed]
- Müller SD, Ziegler JSH, Piegeler T. Local Anesthetics and Recurrence after Cancer Surgery-What's New? A Narrative Review. J Clin Med 2021;10:719. [Crossref] [PubMed]
- Wang HL, Yan HD, Liu YY, et al. Intraoperative intravenous lidocaine exerts a protective effect on cell-mediated immunity in patients undergoing radical hysterectomy. Mol Med Rep 2015;12:7039-44. [Crossref] [PubMed]
- Hou YH, Shi WC, Cai S, et al. Effect of Intravenous Lidocaine on Serum Interleukin-17 After Video-Assisted Thoracic Surgery for Non-Small-Cell Lung Cancer: A Randomized, Double-Blind, Placebo-Controlled Trial. Drug Des Devel Ther 2021;15:3379-90. [Crossref] [PubMed]
- Karcioglu O, Topacoglu H, Dikme O, et al. A systematic review of the pain scales in adults: Which to use? Am J Emerg Med 2018;36:707-14. [Crossref] [PubMed]
- Kavanagh T, Buggy DJ. Can anaesthetic technique effect postoperative outcome? Curr Opin Anaesthesiol 2012;25:185-98. [Crossref] [PubMed]
- Cata JP, Guerra C, Soto G, et al. Anesthesia Options and the Recurrence of Cancer: What We Know so Far? Local Reg Anesth 2020;13:57-72. [Crossref] [PubMed]
- Chraa D, Naim A, Olive D, et al. T lymphocyte subsets in cancer immunity: Friends or foes. J Leukoc Biol 2019;105:243-55. [Crossref] [PubMed]
- Di Vito C, Mikulak J, Zaghi E, et al. NK cells to cure cancer. Semin Immunol 2019;41:101272. [Crossref] [PubMed]
- Wu SY, Fu T, Jiang YZ, et al. Natural killer cells in cancer biology and therapy. Mol Cancer 2020;19:120. [Crossref] [PubMed]
- Cho JS, Lee MH, Kim SI, et al. The Effects of Perioperative Anesthesia and Analgesia on Immune Function in Patients Undergoing Breast Cancer Resection: A Prospective Randomized Study. Int J Med Sci 2017;14:970-6. [Crossref] [PubMed]
- Lim JA, Oh CS, Yoon TG, et al. The effect of propofol and sevoflurane on cancer cell, natural killer cell, and cytotoxic T lymphocyte function in patients undergoing breast cancer surgery: an in vitro analysis. BMC Cancer 2018;18:159. [Crossref] [PubMed]
- Buckley A, McQuaid S, Johnson P, et al. Effect of anaesthetic technique on the natural killer cell anti-tumour activity of serum from women undergoing breast cancer surgery: a pilot study. Br J Anaesth 2014;113:i56-62. [Crossref] [PubMed]
- Huang Y, Ma C, Zhang Q, et al. CD4+ and CD8+ T cells have opposing roles in breast cancer progression and outcome. Oncotarget 2015;6:17462-78. [Crossref] [PubMed]
- Longhini F, Bruni A, Garofalo E, et al. Anesthetic Strategies in Oncological Surgery: Not Only a Simple Sleep, but Also Impact on Immunosuppression and Cancer Recurrence. Cancer Manag Res 2020;12:931-40. [Crossref] [PubMed]
- Zhang C, Xie C, Lu Y. Local Anesthetic Lidocaine and Cancer: Insight Into Tumor Progression and Recurrence. Front Oncol 2021;11:669746. [Crossref] [PubMed]
- Zhang H, Yang L, Zhu X, et al. Association between intraoperative intravenous lidocaine infusion and survival in patients undergoing pancreatectomy for pancreatic cancer: a retrospective study. Br J Anaesth 2020;125:141-8. [Crossref] [PubMed]
- Dubowitz JA, Sloan EK, Riedel BJ. Implicating anaesthesia and the perioperative period in cancer recurrence and metastasis. Clin Exp Metastasis 2018;35:347-58. [Crossref] [PubMed]
(English Language Editor: C. Betlazar-Maseh)