Protective effect of Dachengqi decoction on the pancreatic microcirculatory system in severe acute pancreatitis by down-regulating HMGB-TLR-4-IL-23-IL-17A mediated neutrophil activation by targeting SIRT1
Introduction
Severe acute pancreatitis (SAP), one of the acute abdominals in the clinic, accounts for 10% to 20% of all acute pancreatitis cases (1). Rapid onset characterizes it, rapid progression, and poor prognosis (1). In addition, it causes inflammatory cascades and organ failure in its early stages, resulting in high mortality (1). Therefore, SAP is a serious threat to human health, life, and the economy (1). Despite extensive research and efforts to develop pharmacological tools, there are currently no specific treatments for SAP (1). Therefore, determining the pathophysiological mechanisms underlying SAP is crucial to identify effective therapeutic drugs and preventive measures for SAP.
The pathogenesis of SAP is complex and involves the abnormal activation of pancreatic inflammatory mediators, microcirculation dysfunction, translocation of intestinal flora, apoptosis, and oxidative stress. A large number of latest studies have shown that microcirculation disorder plays an extremely important role in the progression of SAP. It is not only the initiating factor of SAP, but also the factor of sustained and aggravated injury. Acute pancreatitis can damage the pancreas and systemic microcirculation, thus forming a vicious circle (1,2). Additionally, the immune-inflammatory response-mediated pancreatic microcirculation dysfunction is always active, which is also an important molecular biomarker to determine the outcome of pancreatitis (2). In particular, the activation of the inflammatory immune response relies on the recruitment and migration of neutrophils in SAP (3). Therefore, it is an attractive treatment strategy to improve SAP by suppressing the dysfunction mediated by the inflammatory response of the pancreatic microcirculation by promoting neutrophil inactivation.
HMGB1, one of the endogenous molecules secreted from apoptotic and necrotic cells, has a pivotal influence on the development of SAP by activating the immune response (2). Once pancreatic cells are harmfully stimulated, HMGB1 in the nucleus will be modified by acetylation and then released to the extracellular space where it pairs with receptors (TLR-4) on the surface of macrophages to induce TLR-4-mediated immune responses (2,4). This further promotes the release of HMGB1 and amplifies the immune reaction. It is worth noting that HMGB1-TLR-4 signaling stimulates macrophages to secrete the pro-inflammatory cytokine IL-23 and IL-23, promoting Th17, γδ T, NK, and NKT cells to secrete IL-17A to regulate neutrophil activation (5). In addition, the activity and nucleocytoplasmic shuttle of HMGB1 depend on the acetylation degree of HMGB1. SIRT1, one of the key acetylation modifying enzymes, can suppress HMGB1 transcription by deacetylation of HMGB1. Therefore, it is an effective strategy to alleviate the activation mediated by TLR-4 of a neutrophil by triggering SIRT1/HMGB1 signaling in SAP development (6).
Dachengqi decoction (DCQD) is a traditional decoction widely used for SAP treatment (7,8). Its main ingredients include Houpu, Da Huang, Mangxiao, and Zhi Shi (7). DCQD appears positively associated with the improvement of pancreatic microcirculation in SAP (8,9). However, it is a pity that the concrete mechanism that DCQD regulates HMGB1 activation and microcirculation is not clear to date. Therefore, the purpose of the study was to confirm that the improvement of DCQD in pancreatic microcirculation is associated with the suppression of the neutrophil-mediated immune-inflammatory response. However, it promotes the inactivation of the HMGB1-TLR-4-IL-23-IL-17A axis by targeting the SIRT1 signal pathway in SAP. We present the following article in accordance with the ARRIVE reporting checklist (available at https://dx.doi.org/10.21037/gs-21-655).
Methods
Drugs, reagents, instruments, and animals
DCQD consists of Houpu, Da Huang, Mangxiao, and Zhi Shi. The above herbs were acquired from Lv Yuan Pharmaceutical Co., Ltd. in Chengdu. The DCQ granules collected were dissolved in saline to obtain DCQD (2 g/mL). According to the previous article, the concrete, specific compatibility, steps, extraction, and use (9).
Reagents were obtained from the following sources: sodium taurocholate, cerulean (Shanghai Dibao Biology Technology Co., Ltd.); lipase, and amylase, HMGB1, TNF-α, IL-23, IL-1β, KC, IL-17A, LIX, IL-6, and MIP-2 ELISA Kits (Biyuntian); TLR-4, HMGB1 and Acety-HMGB1 antibodies (Abgent, USA); Myeloperoxidase (MPO) and CD68 Colorimetric Activity Assay Kit (BioVision, USA). EX527, r-HMGB1, neutralizing IL-23p19, and IL-17A (Biolegend, San Diego, CA, USA) were purchased from Sigma.
The following instruments were purchased: enzyme labeling instrument, two-dimensional electrophoresis instrument, PCR (Bio-Rad, USA), paraffin embedding and pathological section machines (Leica, Germany), real-time PCR (Applied Biosystems, USA), Western blot imager (Pei Qing, Shang Hai).
Seventy healthy male SD rats (weighing 220±30 g, 6–8 weeks) were obtained from Sichuan University. The rats were located in a standard SPF environment with humidity of 60%±4% and a temperature of 22±2 °C. All animal procedures were approved by the animal care committee of the Sichuan Provincial People’s Hospital (No. 2011220), and the experimental protocols were strictly carried out based on NIH Guidelines for the care and use of animals. A protocol was prepared before the study without registration.
Animal grouping, model establishment, and intervention
The 70 rats were randomly separated into seven groups: Sham, SAP, DCQD, EX527, anti-IL-17, recombinant HMGB1(r-HMGB1), and anti-IL-23p19 groups (n=10). Rats were fed ad libitum for 7 days before establishing the SAP model.
Rats in the SAP, DCQD, EX527, anti-IL-17, r-HMGB1, and anti-IL-23p19 groups were anesthetized with pentobarbital sodium (40 mg/kg). An upper abdominal incision was made by cutting open the skin and muscle to expose the bile duct, temporarily clamped to the small artery at the portal site on the liver. The insulin injection needle was stretched into the biliopancreatic duct by the nipple, and sodium taurocholate (1 mg/kg) got into the biliopancreatic duct by injection. After 2h of injecting sodium taurocholate, the animal in the EX527, DCQD, and r-HMGB1 group were treated with DCQD using an oral gavage at 20 g/kg dose at 2 h after operation (9). Animals in the EX527 and r-HMGB1 group were also treated with EX527 (10 mg/kg) or r-HMGB1 (100 µg/kg) by intraperitoneal injection, according to the previous article (10,11). Rats in the anti-IL-17A groups and the anti-IL-23p19 group were treated with neutralizing antibodies against IL-17A (1 mg/kg) and IL-23p19 (0.2 mg/kg), respectively, by intraperitoneal injection, according to the previous article (12,13). After 12 h, rats in all groups were sacrificed with pentobarbital sodium and serum, and pancreatic tissue was collected for further study.
Cell culture
Rat pancreatic acinar cell lines (AR42J) and RAW264.7 were acquired from ATCC (Rockville, MD, USA). After 30 min of treatment without or with DCQD, AR42J cells were co-incubated with cerulean for 24 h.
Gene silencing
AR42J cells were transfected with control siRNA or TLR-4. The RAW 264.7 cells were intervened with rHMGB1.
TLR4 overexpression
RAW 264.7 cells were designated to transfect TLR4 and control genes using Lipofectamine2000, according to the instructions. TLR4 and IL-23 expression were examined after transfection at 24 h.
Assaying cell viability
MTT was used to evaluate cell survival, and the concrete steps were based on the instruction. AR42J cells were preconditioned with or without DCQD, EX527, or r-HMGB1 (0.004 g/mL), successively 24 co-incubation with cerulean (10 nmol/L).
Microcirculation of the pancreas
Each rat was injected with 1.5 mL of FITC-RBC through the tail vein. We measured flow velocity, functional blood vessel number, red blood cell flow, and blood vessel number to evaluate pancreatic microcirculation using the BI-2000 medical image analysis system.
Histology and wet/weight
After performing hematoxylin and eosin (HE) staining, the excised pancreatic tissue was sectioned and mounted in paraffin, after performing hematoxylin and eosin (HE) staining to evaluate the pathological morphology of the pancreas. The pancreas was assayed under a light microscope. Pancreatic tissue was classified by evaluating necrosis + hemorrhage + edema + inflammation according to the modified Kusske scoring standard blinded by two independent pathologists (9). No inflammatory cell infiltration, hemorrhage, edema and necrosis were scored 0; necrosis area (1–10%), hemorrhage and edema (0–25%) were 1 point; necrosis area (11–20%), hemorrhage and edema (25–50%) were 2 points; necrosis area (21–30%), hemorrhage and edema (50–75%) were 3 points; necrosis area (>30%) hemorrhage and edema (50–55%) were 4 points; 0.5 point for 5 inflammatory cells and 4 points for more than 30 inflammatory cells. The weighted index of the pancreas was calculated as the weight of the pancreas (g)/body weight (g) ×100%.
Amylase and lipase activity assays in serum
The blood collected was centrifuged to obtain the supernatant. The amylase and lipase activity was assayed by exploiting colorimetric activity kits for amylase and lipase, following the manufacturer’s instructions.
Assaying IL-23, HMGB1, IL-1β, IL-17A, MIP-2, TNF-α, LIX, IL-6, and KC
IL-23, HMGB1, IL-1β, IL-17A, MIP-2, TNF-α, LIX, IL-6, and KC concentrations in the pancreas and serum were determined using rat ELISA kits for each protein, following the manufacturer’s instructions.
Detection of IL-23, IL-1β, IL-17A, MIP-2, TNF-α, LIX, IL-6, and KC mRNA expression in the pancreas
Total RNA was extracted using the TRIzol method after homogenizing the frozen pancreas in liquid nitrogen. Real-time PCR was exploited to detect mRNA expression. The concrete steps were in line with the instructions. The primer sequences are displayed in Table 1.
Table 1
Gene | Sense group (5'-3') | Antisense group (5'-3') |
---|---|---|
HMGB1 | ATGGGCAAAGGAGATC | ATTCATCATCATCATCTTCT |
IL-17A | GGAAAGCTGGAC-CACCACA | CACACCCACCAGCATCTTCTC |
IL-23 | AGGACTTGTGCTGTTCTTGTTTTGT | CTCTGGGGTTTGTTTCTTTTCTCTT |
KC | TCACGCTTCTGGGCCTGTTG | CAGCCGACTCATTGGGATCATC |
LIX | TCACGCTTCTGGGCCTGTTG | CAGCCGACTCATTGGGATCATC |
MIP-2 | GGCAAGGCTAACAGACCTGGAAAG | CACATCATCAGGTACGATCCAGGCTTC |
IL-6 | TGCGCTGGGCTTAGATCATT | TGGATGCCTTTTATGTCGTCT |
IL-1β | AGGGAAATCGTGCGTGACAT | GAACCGCTCATTGCCGATAG |
TNF-α | ACCAAGGATGAGGGCGACTA | CAGGCTTATGCCACCACACTT |
β-actin | CATCCGTAAAGACCTCTATGCCAAC | ATGGAGCCACCGATCCACA |
Examining SIRT1 activity
SIRT1 activity was evaluated by the Fluorescent Assay Kit for SIRT1 activity based on instructions.
Western blotting
Total, nuclear, and cytoplasmic proteins were extracted from pancreatic tissues by lysis, centrifugation, and quantification. Western blot analysis was performed using SIRT1 (1:400), Acey-HMGB1 (1:400), TLR-4 (1:1,000), HMGB1 (1:400), β-actin (1:4,000) and Histone (1:1,000). Signals were detected by incubation with a secondary antibody, followed by exposure to the ECL-Plus reagent.
MPO and CD68 assay
Immunohistochemistry was used to evaluate the expression of MPO and CD68 in pancreatic tissues.
Statistical methods
All documents are displayed as mean ± SEM. T-test and one-way ANOVA tests were used to assay multiple comparisons. Tukey’s post-hoc test was exploited to evaluate individual means. P<0.05 was considered significant.
Results
Wet/weight of the pancreas, serum amylase and lipase activity, cell viability, and survival rates
Compared with the SAP group, the wet/weight, activity of amylase and lipase, and mortality in the Sham, DCQD, anti-IL-17A, and anti-IL-23-p19 groups were obliviously decreased (P<0.05). However, with the DCQD group, the wet/weight, activity of amylase and lipase, and the mortality in the markedly increased (P<0.05) (Figure 1A-1C).
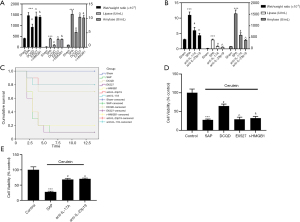
AR42J cells were treated with Cerulean to simulate SAP in rats. Figure 1C,1D shows that cell viability in the Sham, anti-IL-17A, DCQD, and anti-IL-23-p19 groups was significantly up-regulated (P<0.05). Nevertheless, with the DCQD group, cell viability in the EX527 and r-HMGB1 groups was markedly up-regulated (P<0.05) (Figure 1D,1E) (P<0.05).
Pancreatic histopathological morphology and the expression of CD 68 and MPO in the pancreas
HE and immunohistochemistry were used to assess pancreatic histological morphology and CD 68 and MPO expression, respectively. The Sham group showed that the pancreas of some rats had slight edema, a small amount of inflammatory infiltration, but no necrosis or hemorrhage. Pancreatic tissues of the SAP group had severely damaged pancreatic tissue, widespread edema and hemorrhage, a large number of inflammatory cell infiltration, and necrosis of acinar cells with pathological injury scores higher than those of the Sham group (P<0.05). Rat pancreatic tissues in the anti-IL-23-p19, DCQD, and anti-IL-17A groups had edema slightly, a small amount of inflammatory infiltration, hemorrhage, and necrosis with lower pathological injury scores than in the SAP group (P<0.05). Yet with the DCQD group, the r-HMGB1 and EX527 groups had more pancreatic tissue necrosis, inflammatory cell infiltration, edema, and hemorrhage with higher pathological injury scores (P<0.05) (Figure 2).
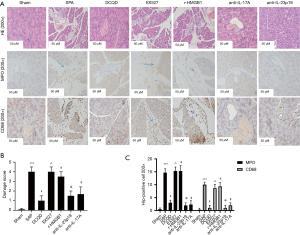
Compared to the sham group, the SAP group has a higher expression of CD68 and MPO (P<0.05). Compared to the SAP group, the DCQD group, the anti-IL-17A, and anti-IL-23-p19 groups have lower expressions of CD68 and MPO (P<0.05). Compared to the DCQD group, EX527 and r-HMGB1 have higher expressions of CD68 and MPO (P<0.05) (Figure 2).
Microcirculatory function of the pancreas
Impaired pancreas microcirculation is a typical clinical manifestation of severe pancreatitis (2,3). Compared to the sham group, the SAP group has a lower blood flow velocity, the number of functional blood vessels, RBC flow, and the number of blood vessels (P<0.05). Compared to the SAP group, the DCQD, anti-IL-17A, and anti-IL-23-p19 groups had blood flow velocity, number of the functional blood vessels, RBC flow, and number of blood vessels (P<0.05). Furthermore, the r-HMGB1 and EX527 groups had a higher blood flow velocity, the number of functional blood vessels, the flow of RBC, and the number of blood vessels than the DCQD group (P<0.05) (Figure 3).
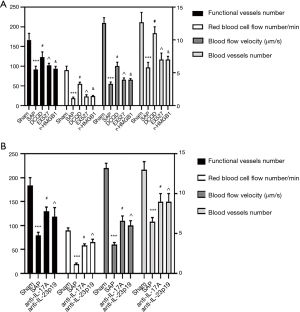
Expression of IL-23, HMGB1, IL-1β, IL-17A, TNF-α and IL-6
The immune-inflammatory response plays a key role in the progression of SAP (2,3). Therefore, the study examined the level of IL-23, HMGB1, IL-1β, IL-17A, TNF-α, and IL-6 in serum and pancreas at both gene and protein levels. The SAP group has a higher level of HMGB1, IL-17A, IL-23, IL-6, TNF-α, and IL-1β in serum and pancreases than the Sham, DCQD, anti-IL-17A, and anti-IL-23-p19 groups (P<0.05). Furthermore, the EX527 and r-HMGB1 groups have higher levels of IL-23, HMGB1, IL-1β, IL-17A, TNF-α, and IL-6 in serum and pancreas at gene and protein levels compared to the DCQD group (P<0.05) (Figure 4).
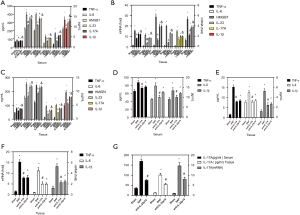
Neutrophil activation
MPO is a surface marker of neutrophils, and MIP-2, LIX, and KC are neutrophil chemokines that reflect neutrophil activation (14). Therefore, we measured the pancreatic MPO activity and the protein and mRNA levels of MIP-2, LIX, and KC in serum and pancreas. The SAP group has higher pancreatic MPO activity and more MIP-2, LIX, and KC expression than the sham group, DCQD, anti-IL-17A, and anti-IL-23-p19 groups (P<0.05). Furthermore, the r-HMGB1 and EX527 groups have higher pancreatic MPO activity and more expression of MIP-2, LIX, and KC compared to the DCQD group (P<0.05) (Figure 5).
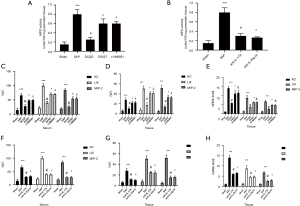
The SIRT1, acety-HMGB1 and TLR-4 expression, HMGB1 translocation, and SIRT1-HMGB1combination in the pancreas
The HMGB1-TLR4 signal pathway significantly influences SAP, and SIRT1 regulates HMGB1 can activation through deacetylation (7,8). Therefore, we examined the activity of SIRT1, the combination of SIRT1 and HMGB1, and the level of SIRT1, acety-HMGB1, TLR4, and the mitigation of HMGB1 from nuclear to the cytoplasm in vitro and vivo. The SAP group had a higher expression of acety-HMGB1, TLR-4, and cytoplasmic HMGB1 than the sham group with lower SIRT1 activity, lower level of nuclear HMGB1, SIRT1, and lower combination of SIRT1-HMGB1 (P<0.05), while the DCQD group showed an increase in SIRT1 activity, a combination of SIRT1 and HMGB1, and a level of SIRT1 and nuclear HMGB1 with a decrease in the level of acety-HMGB1, TLR-4, and cytoplasmic HMGB1 than the SAP group (P<0.05). Furthermore, the EX527 group showed a higher level of acety-HMGB1, TLR-4, and HMGB1 in the cytoplasm than the DCQD group with a lower combination of SIRT1-HMGB1, lower expression of SIRT1 and nuclear HMGB1, and lower activity of SIRT1 (P<0.05) (Figure 6).
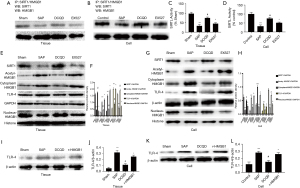
The release of IL-23, though relying on the HMGB1/TLR-4 way in SAP
As shown in Figure 7A,7B, r-HMGB1 up-regulated IL-23 secretion and increased the IL-23 and TLR-4 levels (P<0.5). Compared to the SAP group, the DCQD and TLR-4 siRNA groups have lower IL-23 and TLR-4. However, overexpression of TLR-4 can up-regulate IL-23 and TLR-4 expression using a TLR-4 expression plasmid, demonstrating higher expression of IL-23 and TLR-4 in the TLR-4 plasmid group than the SAP group (P<0.05).
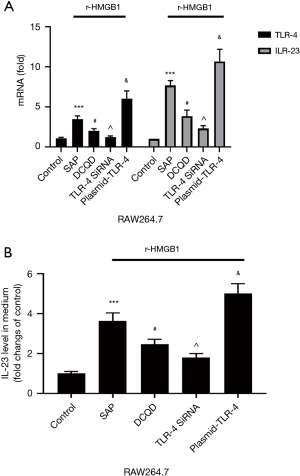
The DCQD signal pathway in the pancreatic microcirculatory system in SAP (Figure 8)
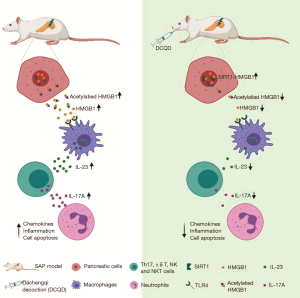
DCQD treatment improves SAP-induced pancreatic microcirculatory dysfunction by inhibiting neutrophil-mediated inflammation via inactivating HMGB1-TLR-4-IL-23-IL-17A signaling by targeting SIRT1.
Discussion
One common clinical critical illness, SAP, easily caused multiple organ failure and frequent death (1). This study used sodium taurodeoxycholate or cerulean to induce SAP in vivo and in vitro, regardless. In vivo, SAP caused a disturbance in the microcirculation of the pancreas, an increase in serum amylase and lipase activity, and an increase in the wet weight ratio of the pancreas. In vitro, cerulean can significantly decrease AR42J cell viability, which is consistent with earlier studies (9). These indicated that SAP in vivo and in vitro models was successfully established. We also showed that DCQD, anti-IL-17A, or anti-IL-23-p19 treatment remarkably decreased lipase and amylase activity, reduced microcirculatory pancreatic pathological injury and dysfunction, downregulated the weight index, and improved cerulean -induced the decline in the cell viability of AR42J.
Furthermore, both r-HMGB1 (an HMGB1 agonist) and EX527 (SIRT1 inhibitor) effectively abated the protective effect of DCQD in SAP. The findings were supported, as they showed a higher serum activity of lipase and amylase, more serious pancreatic pathological injury, weight index and cell viability, and worse microcirculatory function in the EX527 and r-HMGB1 groups than in the DCQD group. Furthermore, previous studies have confirmed that activating the HMGB1 and IL-23/IL-17A pathways significantly aggravated pancreatic microcirculatory impairment. Therefore, our study further confirms that the protective effect DCQD on SAP was achieved through down-regulation of HMGB1-mediated pancreatic microcirculatory dysfunction, and the IL-23/IL-17A signal pathway plays an axis in regulating microcirculatory dysfunction in SAP.
Increasing evidence showed that the inflammatory response significantly influences TNF-α, IL-6, and IL-1β are the most important symbol of inflammatory cytokines in SAP (14). TNF-α is an inflammation-initiating factor that can mediate the release of IL-6 and other inflammatory cytokines, stimulate oxygen free radicals and nitric oxide production, promote leukocyte chemotaxis and adhesion, and damage pancreatic tissue (15). IL-6 has a critical influence in initiating an immune response in SAP, and is released primarily by macrophages, T lymphocytes, dendritic cells, and T cells (14,15). IL-1β is deeply involved in the destruction of pancreatic tissue and the formation of edema in SAP (15). In this study, the intervention with anti-IL-17A, DCQD, or anti-IL-23p19 significantly decreased TNF-α, IL-6, and IL-1β expression. However, r-HMGB1 and EX527 can eliminate the influence of DCQD on the inflammatory response in SAP, as confirmed higher expression of TNF-α, IL-6, and IL-1β in the r-HMGB1 and EX527 groups than in the DCQD group. The above results implied that DCQD could reduce SAP-induced microcirculatory dysfunction by inhibiting the HMGB1-mediated inflammatory response, and the IL-23/IL-17A signal pathway can improve microcirculatory dysfunction by attenuating inflammation in SAP.
Interestingly, blocking neutrophil activation has previously been demonstrated to suppress the inflammatory response in SAP (7). Therefore, the intensity of the inflammatory response depends on the activation of neutrophils in SAP. In addition, MPO is a surface marker of neutrophils, and KC, LIX, and MIP-2 are neutrophil chemokines that reflect neutrophil activation by recruitment and migration of neutrophils. Treatment with anti-IL-23-p19, DCQD, or anti-IL-17A decreased pancreatic MPO expression, activity, and MIP-2., KC, and LIX. However, the r-HMGB1 intervention can reduce the negative influence of DCQD on neutrophil inactivation and lessen neutrophil chemokines, as it showed higher MPO activity and higher expression of MIP-2, KC, and LIX in the r-HMGB1 group than in the DCQD group. These findings further demonstrated that anti-IL-23-p19, DCQD, or anti-IL-17A reduce the inflammatory response by activating neutrophils in SAP.
IL-17A is a common molecule that critically modulates host defense against detrimental inflammatory stimulation (6). Controlling neutrophil activation and recruitment to the pancreas is the main mechanism of IL-17A activity (7). IL-17A is mainly released from NKT cells, γδ T cells, Th17 cells, etc. (6,15). In particular, the release of IL-17A is controlled by the heterodimeric cytokine IL-23 through the following mechanism (16,17): IL-23 triggers naïve CD4+ T cells differentiation into Th17 and subsequently stimulates NKT cells to release IL-17A, along with anti-CD3. Furthermore, IL-23 promotes the release of IL-17A from γδ T cells by working with IL-1 (17). In our study, SAP can stimulate the activation of IL-23/IL-17A signaling, and DCQD significantly promoted inactivation of the IL-23/IL-17A axis, as it showed a lower level of IL-17A and IL-23 in the DCQD group than in the SAP group. However, r-HMGB1 can boost activating IL-23/IL-17A signaling in SAP, demonstrating higher IL-17A and IL-23 levels in an r-HMGB1 group than the DCQD group. Furthermore, we also demonstrated that neutralizing IL-23 reduced the expression of IL-17A in SAP, which further confirmed that IL-23 could promote IL-17A secretion. These results were in accordance with previous research that implied that the inhibitory effect of DCQD in neutrophil inactivation is through the inactivation of the IL-23/IL-17A axis through HMGB1.
HMGB1 is a non-DNA binding protein in the nucleus of eukaryotic cells and is widely distributed in the pancreas, lung, liver, kidney, lymph, and other tissues (2). It is known to function as a DAMP, interacting with members of RAGE and TLR (2). In our study, SAP only influences TLR-4. This is inconsistent with previous research attributed to drug dosage, purity, technology, and individual differences in rats. TLR-4 is a pattern-recognition receptor (PRR), and it has been extensively validated that TLR-4 mediates HMGB1-induced pancreatic injury in SAP (2). Previous research showed that HMGB1 stimulates the expression of TLR-4 in the pancreas, which is positively related to promoting macrophages and dendritic cells to secrete IL-23 in SAP (8). Blocking TLR-4 can trigger the inactivation of IL-23/IL-17A signaling (18). In our in vivo study, DCQD treatment markedly advanced the inactivation of the HMGB1/TLR-4 signal pathway, as shown by a lower expression of TLR-4 and CD68, and lower acetylation, migration, and release of HMGB1. Furthermore, r-HMGB1 can abolish the impact of DCQD on the signal pathway of HMGB1/TLR-4 in SAP, as confirmed higher expression of TLR-4 and CD68 and greater acetylation, migration, and release of HMGB1 in the r-HMGB1 group than in the DCQD group.
In vitro studies further confirmed that DCQD could decrease HMGB1 secretion, and HMGB1 can promote macrophages to secrete IL-23 and increase IL-23 and TLR-4 expression. In addition, SiRNA TLR-4 can advance macrophage inactivation and alleviate IL-23 and TLR-4 expression. However, overexpression of TLR-4 has the opposite influence, as confirmed higher expression of LR-4 and IL-23 in the plasmid group than in the SAP group. The above results indicated that the negative effect of DCQD on the activation of IL-23/IL-17A signaling is through the promotion of the inactivation of the HMGB1-TLR-4 signal pathway through deacetylation of HMGB1.
Noteworthy, the degree of deacetylation of HMGB1 is dependent on SIRT1, one of NAD + dependent histone deacetylase, which can deacetylate the activities of HMGB1 through interacting with HMGB1 at promoter region (19). In the study, DCQD can suppress the sodium taurocholate or cerulean-induced decrease in SIRT1 activity, expression, and interaction of HMGB1. However, EX527 can eliminate the influence of DCQD on the SIRT-HMGB1 axis in SAP. This indicated that DCQD could alleviate HMGB1 acetylation by regulating the SIRT-HMGB1 signaling pathway in SAP.
In conclusion, our study provides compelling evidence that the protective effect of DCQD on the pancreatic microcirculatory system in SAP is through inhibiting neutrophil-mediated inflammatory reactions. The above action mechanism of DCQD is likely associated with the inactivation of the HMGB1-TLR-4-IL-23-IL-17A axis by targeting SIRT1. However, this study was only performed on non-knockout mice with small sample size. More studies are required with larger sample sizes, knockout mice and clinical observations, and more cellular intervention to confirm the efficacy and mechanism of DCQD in SAP.
Acknowledgments
Funding: The study was funded by the Foundation of Sichuan Provincial People’s Hospital (No. 2020LY07, No. 2017LY11), and scientific research project of Sichuan Provincial Department of Science and Technology (No. 2020YJ0179).
Footnote
Reporting Checklist: The authors have completed the ARRIVE reporting checklist. Available at https://dx.doi.org/10.21037/gs-21-655
Data Sharing Statement: Available at https://dx.doi.org/10.21037/gs-21-655
Conflicts of Interest: All authors have completed the ICMJE uniform disclosure form (available at https://dx.doi.org/10.21037/gs-21-655). Dr. JW reported that the study was funded by the Foundation of Sichuan Provincial People’s Hospital (No. 2017LY11). Dr. JZ reported that the study was funded by the Foundation of Sichuan Provincial People’s Hospital (No. 2020LY07) and scientific research project of Sichuan Provincial Department of Science and Technology (No. 2020YJ0179). The other authors have no conflicts of interest to declare.
Ethical Statement: The authors are accountable for all aspects of the work in ensuring that questions related to the accuracy or integrity of any part of the work are appropriately investigated and resolved. All animal procedures were approved by the animal care committee of the Sichuan Provincial People’s Hospital (No. 2011220), and the experimental protocols were strictly carried out based on NIH Guidelines for the care and use of animals.
Open Access Statement: This is an Open Access article distributed in accordance with the Creative Commons Attribution-NonCommercial-NoDerivs 4.0 International License (CC BY-NC-ND 4.0), which permits the non-commercial replication and distribution of the article with the strict proviso that no changes or edits are made and the original work is properly cited (including links to both the formal publication through the relevant DOI and the license). See: https://creativecommons.org/licenses/by-nc-nd/4.0/.
References
- Wu BU, Johannes RS, Sun X, et al. The early prediction of mortality in acute pancreatitis: a large population-based study. Gut 2008;57:1698-703. [Crossref] [PubMed]
- Broggi A, Granucci F. Microbe- and danger-induced inflammation. Mol Immunol 2015;63:127-33. [Crossref] [PubMed]
- Leppkes M, Maueröder C, Hirth S, et al. Externalized decondensed neutrophil chromatin occludes pancreatic ducts and drives pancreatitis. Nat Commun 2016;7:10973. [Crossref] [PubMed]
- Shen X, Li WQ. High-mobility group box 1 protein and its role in severe acute pancreatitis. World J Gastroenterol 2015;21:1424-35. [Crossref] [PubMed]
- Yan B, Chen F, Xu L, et al. HMGB1-TLR4-IL23-IL17A axis promotes paraquat-induced acute lung injury by mediating neutrophil infiltration in mice. Sci Rep 2017;7:597. [Crossref] [PubMed]
- Ni J, Hu G, Xiong J, et al. Involvement of interleukin-17A in pancreatic damage in rat experimental acute necrotizing pancreatitis. Inflammation 2013;36:53-65. [Crossref] [PubMed]
- Zhang MJ, Zhang GL, Yuan WB, et al. Treatment of abdominal compartment syndrome in severe acute pancreatitis patients with traditional Chinese medicine. World J Gastroenterol 2008;14:3574-8. [Crossref] [PubMed]
- Chen Z, Chen Y, Pan L, et al. Dachengqi Decoction Attenuates Inflammatory Response via Inhibiting HMGB1 Mediated NF-κB and P38 MAPK Signaling Pathways in Severe Acute Pancreatitis. Cell Physiol Biochem 2015;37:1379-89. [Crossref] [PubMed]
- Wang J, Chen G, Gong H, et al. Amelioration of experimental acute pancreatitis with Dachengqi Decoction via regulation of necrosis-apoptosis switch in the pancreatic acinar cell. PLoS One 2012;7:e40160 [Crossref] [PubMed]
- Huang J, Tian R, Yang Y, et al. The SIRT1 inhibitor EX-527 suppresses mTOR activation and alleviates acute lung injury in mice with endotoxiemia. Innate Immun 2017;23:678-86. [Crossref] [PubMed]
- Li G, Wu X, Yang L, et al. TLR4-mediated NF-κB signaling pathway mediates HMGB1-induced pancreatic injury in mice with severe acute pancreatitis. Int J Mol Med 2016;37:99-107. [Crossref] [PubMed]
- Hu X, Ma R, Lu J, et al. IL-23 Promotes Myocardial I/R Injury by Increasing the Inflammatory Responses and Oxidative Stress Reactions. Cell Physiol Biochem 2016;38:2163-72. [Crossref] [PubMed]
- Sun J, Zhang S, Zhang X, et al. IL-17A is implicated in lipopolysaccharide-induced neuroinflammation and cognitive impairment in aged rats via microglial activation. J Neuroinflammation 2015;12:165. [Crossref] [PubMed]
- Kolaczkowska E, Kubes P. Neutrophil recruitment and function in health and inflammation. Nat Rev Immunol 2013;13:159-75. [Crossref] [PubMed]
- Xu P, Wang J, Yang ZW, et al. Regulatory roles of the PI3K/Akt signaling pathway in rats with severe acute pancreatitis. PLoS One 2013;8:e81767 [Crossref] [PubMed]
- Cua DJ, Tato CM. Innate IL-17-producing cells: the sentinels of the immune system. Nat Rev Immunol 2010;10:479-89. [Crossref] [PubMed]
- Zheng Y, Danilenko DM, Valdez P, et al. Interleukin-22, a T(H)17 cytokine, mediates IL-23-induced dermal inflammation and acanthosis. Nature 2007;445:648-51. [Crossref] [PubMed]
- Wang X, Sun R, Wei H, et al. High-mobility group box 1 (HMGB1)-Toll-like receptor (TLR)4-interleukin (IL)-23-IL-17A axis in drug-induced damage-associated lethal hepatitis: Interaction of γδ T cells with macrophages. Hepatology 2013;57:373-84. [Crossref] [PubMed]
- Wei S, Gao Y, Dai X, et al. SIRT1-mediated HMGB1 deacetylation suppresses sepsis-associated acute kidney injury. Am J Physiol Renal Physiol 2019;316:F20-31. [Crossref] [PubMed]