Long noncoding RNA SNHG6 promotes papillary thyroid cancer cells proliferation via regulating miR-186/CDK6 axis
Introduction
In recent years, the incidence rate of thyroid cancer has been increasing and becoming the most common endocrine malignancy (1,2). Papillary thyroid cancer (PTC) is the most common subtype, accounting for about 80% of all thyroid cancers (3). Although the degree of malignancy of PTC is low and the prognosis is usually good, many PTC patients will have tumor recurrence after operation (4,5). Moreover, the prognosis of advanced PTC is still not ideal (4,5). The pathogenesis of PTC is not completely clear, although many risk factors are known to be closely related to the occurrence and development of PTC, such as genetic factors, environmental exposure, and epigenetic changes (6,7). Therefore, it is of great significance to study the molecular mechanism of PTC progression for finding new therapeutic targets.
Long noncoding RNAs (lncRNAs) have a length of >200 nucleotides, and participate in cell biological processes through a variety of regulatory ways (8). Many studies have shown that lncRNAs play a critical role in the occurrence and development of tumors (9). In general, lncRNAs are involved in tumor proliferation, metastasis, differentiation, metabolism, and angiogenesis by regulating key tumor suppressor genes or oncogenes (10,11). There are many reports about lncRNAs in PTC. For example, lncRNA GAS8-AS1 is downregulated in PTC tissues and cell lines. Its low expression is associated with higher TNM stage and lymph node metastasis. GAS8-AS1 can upregulate the expression of ATG5 and ATG7 through miR-187-3p and miR-1343-3p, which significantly promote autophagy and inhibit the proliferation of PTC cells and tumorigenesis (12). Small nucleolar RNA host gene 15 (SNHG15) is highly expressed in PTC. As a competitive endogenous RNA, it regulates the YAP1-Hippo signaling pathway by binding miR-200a-3p, thus promoting the occurrence and development of PTC (13).
SNHG6 is a newly discovered lncRNAs that is highly expressed and plays a carcinogenic role in many human malignant tumors, such as lung cancer, colorectal cancer, hepatocellular carcinoma, and gastric cancer (14-18). However, the role of SNHG6 in PTC remains to be studied, and its expression, biological function and molecular mechanism in PTC are still unclear. So we explored the role of SNHG6 in the progress of PTC and its regulatory mechanism.
We present the following article in accordance with the MDAR reporting checklist (available at https://dx.doi.org/10.21037/gs-21-586).
Methods
Bioinformatics analysis
This study used the Starbase database (http://starbase.sysu.edu.cn) and the UALCAN database (http://ualcan.path.uab.edu/index.html) to perform a bioinformatics analysis, thus identifying the expression differences of SNHG6 and miR-186 in thyroid cancer samples and normal tissues. The study was conducted in accordance with the Declaration of Helsinki (as revised in 2013).
Cell culture and transfection
Nthy-ori 3-1 and PTC cell lines (TPC-1 and K1) were purchased from American Type Culture Collection (VA, USA). All cell lines used in the study were cultured in Dulbecco’s modified Eagle’s medium (Gibco, MD, USA) containing 10% fetal bovine serum (Hyclone, UT, USA). The cell incubator was set at 37 °C with 5% CO2. According to the manufacturer’s instructions, Lipofectamine 3000 reagent (Invitrogen, Waltham, MA, USA) was used to transfect PTC cells.
RNA extraction and quantitative real-time PCR (qRT-PCR)
Trizol reagent (Invitrogen) was used to extract total RNA from cultured cells, and the purity and concentration of RNA were determined by spectrophotometer. Using PrimeScript RT reagent kit (Takara, Dalian, China), 1 µg RNA was reversely transcribed into cDNA. SYBR Green Premix EX Taq (Takara) and specific primers were used for the PCR reaction. β-actin was used as an internal reference. The relative expression level of mRNA was calculated by the 2−∆∆Ct method. The primer sequence was as follows: SNHG6-F, 5'-ATACTTCTGCTTCGTTACCT-3'; SNHG6-R, 5'-CTCATTTTCATCATTTGCT-3'; β-actin-F, 5'-AGCGAGCATCCCCCAAAGTT-3'; β-actin-R, 5'-GGGCACGAAGGCTCATCATT-3'.
Cell counting kit-8 (CCK-8) assay
A total of 3,000 transfected PTC cells were seeded in 96-well plates. At the designated time point, 10 µL CCK-8 solution (Beyotime, Shanghai, China) was added to each well. After incubation for 1 h, the optical density value at 450 nm was detected by spectrophotometer (Bio-Rad, Hercules, CA, USA).
Colony formation assay
The transfected cells were seeded in a 6-well plate with a density of 500 cells per well. After 2 weeks of culture, the cells were fixed with 4% paraformaldehyde for 30 min, and stained with 0.5% crystal violet for 15 min. The images were photographed, and the number of cell clones was counted.
5-ethynyl-2’-deoxyuridine (EdU) assay
After transfection, a BeyoClickTM EdU Cell Proliferation Kit with Alexa Fluor 594 (Beyotime) was used to detect PTC cells’ proliferation. The cells were photographed by fluorescence microscope (Olympus, Tokyo, Japan) [EdU—red fluorescence, 4’,6-diamidino-2-phenylindole (DAPI)—blue fluorescence] to calculate the positive rate.
Luciferase reporter assay
The SNHG6 or CDK6 3'UTR sequence of the wild-type (WT) or mutation (MUT) containing the miR-186 binding site were cloned into pGLOvector (Promega, Madison, WI, USA). miR-186 mimics and luciferase reporter plasmid were cotransfected into the cells and cultured for 48 h. The luciferase activity was detected by a dual-luciferase reporter assay system (Promega).
Protein extraction and western blot
The total protein was extracted from the cells with RIPA lysis buffer containing protease inhibitor. The protein concentration was determined by the bicinchoninic acid (BCA) method. Next, sodium dodecyl sulfate-polyacrylamide gel electrophoresis (SDS-PAGE) was used to separate the total protein and transfer the protein to the polyvinylidene fluoride (PVDF) membrane, which was blocked with 5% skim milk for 2 h at room temperature. After that, the membrane was mixed with anti-CDK6 antibody (ab124821, Abcam, USA), and anti-β-actin antibody (ab8226, Abcam) was incubated overnight at 4 °C. After washing, the membrane was incubated with Rabbit anti-Human IgG H&L (HRP) (ab6759, Abcam, USA) at room temperature for 1 h. The protein bands were detected by NovexTM ECL Chemiluminescent Substrate Reagent Kit (Thermo Fisher Scientific, Inc., Waltham, MA, USA).
Statistical analysis
The data were analyzed by GraphPad Prism 5.0 software (GraphPad, CA, USA). Each experiment was repeated at least three times independently, and the data were expressed as mean ± standard deviation (SD). Independent sample t-test was used to compare the differences between groups. One-way analysis of variance (ANOVA) and Bonferroni post-test were used to analyze the differences among three groups or more. P value <0.05 was statistically significant.
Results
Expression of SNHG6 in PTC
Using the UALCAN database, we found that the expression of SNHG6 in thyroid cancer tissues was significantly higher than in normal tissues (Figure 1A), and its expression was closely related to tumor stage (Figure 1B). Moreover, the expression of SNHG6 in PTC cell lines TPC-1 and K1 was significantly higher than in Nthy-ori 3-1 cells (Figure 1C). These results indicated that SNHG6 is highly expressed in PTC. Subsequently, we overexpressed SNHG6 in TPC-1 cells and knocked down SNHG6 in K1 cells (Figure 1D,1E).
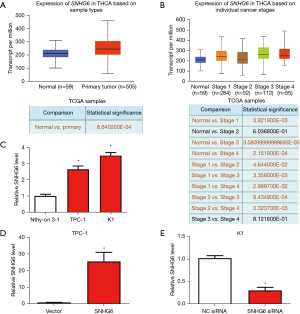
Effect of SNHG6 on proliferation of PTC cells
We studied the effect of SNHG6 on the proliferation of PTC cells. The results of the CCK-8 experiment showed that overexpression of SNHG6 significantly increased the activity of TPC-1 cells, and silencing SNHG6 inhibited the activity of K1 cells (Figure 2A,2B). The colony formation assay showed that overexpression of SNHG6 significantly enhanced the colony formation ability of TPC-1 cells, and silencing SNHG6 inhibited the colony formation ability of K1 cells (Figure 2C,2D). The EdU assay showed that overexpression of SNHG6 significantly promoted the proliferation of TPC-1 cells, and silencing SNHG6 inhibited the proliferation of K1 cells (Figure 2E,2F). These results indicated that SNHG6 can promote the proliferation of PTC cells.
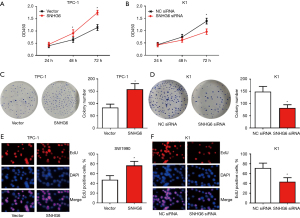
Downstream target of SNHG6
Using the Starbase database (http://starbase.sysu.edu.cn), we predicted a potential miR-186 binding site in SNHG6 (Figure 3A). Subsequently, we used a luciferase reporter gene experiment to verify this. The results showed that miR-186 mimics could significantly inhibit the luciferase activity of the plasmid containing the WT SNHG6 sequence but did not affect the mutant plasmid (Figure 3B). These results indicated that miR-186 can bind to SNHG6 and is the downstream target of SNHG6. Moreover, using the UALCAN database, we found that the expression of miR-186 in thyroid cancer tissues was significantly lower than in normal tissues (Figure 3C). Moreover, the expression of miR-186 in the PTC cell lines TPC-1 and K1 was significantly lower than in Nthy-ori 3-1 cells (Figure 3D).
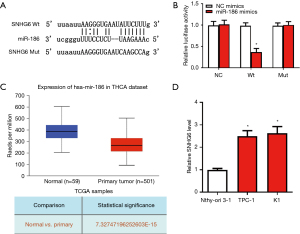
Mechanism of SNHG6 regulation of PTC cell proliferation
To investigate the mechanism of SNHG6 enhancing the proliferation of PTC cells, we transfected miR-186 mimics into SNHG6-overexpressing TPC-1 cells, and miR-186 inhibitor into SNHG6 knockdown K1 cells. CCK-8, colony formation, and EdU experiments showed that miR-186 mimics could reverse the proliferation of TPC-1 cells induced by overexpression of SNHG6, and miR-186 inhibitor partially eliminated the inhibition of proliferation induced by silencing SNHG6 (Figure 4). These results indicated that SNHG6 regulates the proliferation of PTC cells through miR-186.
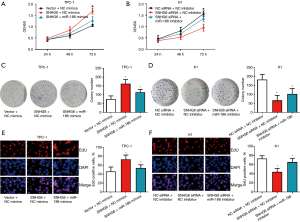
Mechanism of SNHG6 promotion of CDK6 expression
Using the Starbase database, we predicted a potential miR-186 binding site in the 3'UTR sequence of CDK6 (Figure 5A), suggesting that it may be a downstream target gene of miR-186. We used a luciferase reporter gene experiment to verify this. The results showed that miR-186 mimics could significantly inhibit the luciferase activity of the plasmid containing the WT CDK6 3'UTR sequence, but did not affect the mutant plasmid (Figure 5B). Western blot analysis showed that overexpression of miR-186 significantly inhibited the expression of CDK6 protein, and knockdown of miR-186 promoted the expression of CDK6 protein (Figure 5C,5D). These results indicated that miR-186 can target CDK6 3'UTR and inhibit the expression of CDK6 protein. Furthermore, we found that overexpression of SNHG6 promoted the expression of CDK6 protein, whereas miR-186 mimics reversed this effect (Figure 5E). Similarly, silencing SNHG6 inhibited the expression of CDK6, and miR-186 inhibitor reversed this effect (Figure 5F). These results indicated that SNHG6 promotes the expression of CDK6 by regulating miR-186.
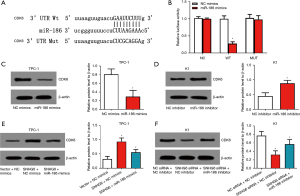
Discussion
Thyroid cancer is the most common cancer in the endocrine system. With the deepening of research, many molecular pathogenesis of thyroid cancer have been discovered. For example, RAS mutations, BRAF mutations, and activation of the mitogen-activated protein kinase pathway play an important role in tumorigenesis (18). Moreover, some molecular markers, such as TERT promoter mutation and TP53 mutation, are closely related to tumor progression (18).
In recent years, miRNAs and lncRNAs have been confirmed to play a crucial role in the occurrence and development of various cancers, and may be effective targets for the treatment of malignant tumors (19,20). In this study, we explored the important role of lncRNA SNHG6 in the malignant process of PTC through regulating miR-186/CDK6, and we determined that SNHG6 is a carcinogen in PTC.
First, bioinformatics analysis revealed SNHG6 was highly expressed in PTC tissue, and its expression was closely related to the tumor stage of PTC. We also confirmed that SNHG6 was highly expressed in a PTC cell line. The expression and clinical significance of SNHG6 in many types of tumor have been reported, and it is suggested that the high expression of SNHG6 in a tumor is related to malignant phenotype and poor clinical prognosis of the tumor (14-17,19). The expression of SNHG6 in PTC and its clinical significance are revealed for the first time.
In addition, we found that overexpression of SNHG6 promoted proliferation of PTC cells, which was inhibited by silencing SNHG6. The biological role of SNHG6 as an oncogene has been reported in other tumors (14-17,19), but for the first time in PTC. Our mechanism studies showed that SNHG6 can bind to miR-186 and affect its function, thus regulating the proliferation of PTC cells. lncRNA miRNA mRNA competitive endogenous RNA regulatory network is a common molecular regulatory mechanism for lncRNA (21,22). In this mode, lncRNAs can competitively bind miRNAs with mRNAs, thus affecting the inhibitory effect of miRNAs on mRNA translation and ultimately promoting mRNA translation (21,22). In this study, we demonstrated that SNHG6 promotes the expression of CDK6 by binding to miR-186.
The expression of miR-186 is low in many tumors, such as colorectal cancer, liver cancer, breast cancer, osteosarcoma, and esophageal cancer. It is a tumor suppressor gene that inhibits malignant progression (23-27). Those studies also found many target genes of miR-186, such as SMAD6/7, PTPN11, ZEB1, GPRC5A, PARP9, etc., indicating that miR-186 plays a biological role by inhibiting the expression of these genes (23-27). In this study, we found a new target gene of miR-186, viz., CDK6. CDK6 is a cell cycle-dependent kinase and a transcription regulator, which plays a vital role in G1 phase progression and G1/S cell cycle transition (28-30). Studies have shown that it is highly expressed in many malignant tumors and promotes the proliferation of tumor cells (28-30). Inhibitors targeting CDK6 can slow down tumor growth (28-30). In this study, we confirmed that SNHG6 promotes the expression of CDK6 by regulating miR-186. Therefore, we believe that SNHG6 promotes cell proliferation by regulating the miR-186/CDK6 axis in PTC. Moreover, if a targeted drug is designed for SNHG6 as a target, it may have potential application value in the clinical treatment of PTC, and it has potential significance for improving the prognosis of PTC.
In conclusion, SNHG6 was highly expressed in PTC, and miR-186 was minimally expressed in PTC. SNHG6 can promote the proliferation of PTC cells by regulating the miR-186/CDK6 axis, which is expected to become a potential therapeutic target for PTC.
Acknowledgments
Funding: None.
Footnote
Reporting Checklist: The authors have completed the MDAR reporting checklist. Available at https://dx.doi.org/10.21037/gs-21-586
Data Sharing Statement: Available at https://dx.doi.org/10.21037/gs-21-586
Conflicts of Interest: Both authors have completed the ICMJE uniform disclosure form (available at https://dx.doi.org/10.21037/gs-21-586). The authors have no conflicts of interest to declare.
Ethical Statement: The authors are accountable for all aspects of the work in ensuring that questions related to the accuracy or integrity of any part of the work are appropriately investigated and resolved. The study was conducted in accordance with the Declaration of Helsinki (as revised in 2013).
Open Access Statement: This is an Open Access article distributed in accordance with the Creative Commons Attribution-NonCommercial-NoDerivs 4.0 International License (CC BY-NC-ND 4.0), which permits the non-commercial replication and distribution of the article with the strict proviso that no changes or edits are made and the original work is properly cited (including links to both the formal publication through the relevant DOI and the license). See: https://creativecommons.org/licenses/by-nc-nd/4.0/.
References
- Miranda-Filho A, Lortet-Tieulent J, Bray F, et al. Thyroid cancer incidence trends by histology in 25 countries: a population-based study. Lancet Diabetes Endocrinol 2021;9:225-34. [Crossref] [PubMed]
- Ho AS, Luu M, Barrios L, et al. Incidence and mortality risk spectrum across aggressive variants of papillary thyroid carcinoma. JAMA Oncol 2020;6:706-13. [Crossref] [PubMed]
- Shen X, Zhu G, Liu R, et al. Patient age-associated mortality risk is differentiated by BRAF V600E status in papillary thyroid cancer. J Clin Oncol 2018;36:438-45. [Crossref] [PubMed]
- Dehbi HM, Mallick U, Wadsley J, et al. Recurrence after low-dose radioiodine ablation and recombinant human thyroid-stimulating hormone for differentiated thyroid cancer (HiLo): long-term results of an open-label, non-inferiority randomised controlled trial. Lancet Diabetes Endocrinol 2019;7:44-51. [Crossref] [PubMed]
- Hung ML, Wu JX, Li N, et al. Association of radioactive iodine administration after reoperation with outcomes among patients with recurrent or persistent papillary thyroid cancer. JAMA Surg 2018;153:1098-104. [Crossref] [PubMed]
- Lee SH, Roh JL, Gong G, et al. Risk factors for recurrence after treatment of N1b papillary thyroid carcinoma. Ann Surg 2019;269:966-71. [Crossref] [PubMed]
- Kitahara CM, Slettebø Daltveit D, Ekbom A, et al. Maternal health, in-utero, and perinatal exposures and risk of thyroid cancer in offspring: a Nordic population-based nested case-control study. Lancet Diabetes Endocrinol 2021;9:94-105. [Crossref] [PubMed]
- Winkle M, El-Daly SM, Fabbri M, et al. Noncoding RNA therapeutics - challenges and potential solutions. Nat Rev Drug Discov 2021;20:629-51. [Crossref] [PubMed]
- Liu SJ, Dang HX, Lim DA, et al. Long noncoding RNAs in cancer metastasis. Nat Rev Cancer 2021;21:446-60. [Crossref] [PubMed]
- Goodall GJ, Wickramasinghe VO. RNA in cancer. Nat Rev Cancer 2021;21:22-36. [Crossref] [PubMed]
- Rinn JL, Chang HY. Long noncoding RNAs: molecular modalities to organismal functions. Annu Rev Biochem 2020;89:283-308. [Crossref] [PubMed]
- Qin Y, Sun W, Wang Z, et al. ATF2-induced lncRNA GAS8-AS1 promotes autophagy of thyroid cancer cells by targeting the miR-187-3p/ATG5 and miR-1343-3p/ATG7 axes. Mol Ther Nucleic Acids 2020;22:584-600. [Crossref] [PubMed]
- Wu DM, Wang S, Wen X, et al. LncRNA SNHG15 acts as a ceRNA to regulate YAP1-Hippo signaling pathway by sponging miR-200a-3p in papillary thyroid carcinoma. Cell Death Dis 2018;9:947. [Crossref] [PubMed]
- Geng H, Li S, Xu M. Long noncoding RNA SNHG6 functions as an oncogene in non-small cell lung cancer via modulating ETS1 signaling. Onco Targets Ther 2020;13:921-30. [Crossref] [PubMed]
- Wang X, Lan Z, He J, et al. LncRNA SNHG6 promotes chemoresistance through ULK1-induced autophagy by sponging miR-26a-5p in colorectal cancer cells. Cancer Cell Int 2019;19:234. [Crossref] [PubMed]
- Chang L, Yuan Y, Li C, et al. Upregulation of SNHG6 regulates ZEB1 expression by competitively binding miR-101-3p and interacting with UPF1 in hepatocellular carcinoma. Cancer Lett 2016;383:183-94. [Crossref] [PubMed]
- Yan K, Tian J, Shi W, et al. LncRNA SNHG6 is associated with poor prognosis of gastric cancer and promotes cell proliferation and EMT through epigenetically silencing p27 and sponging miR-101-3p. Cell Physiol Biochem 2017;42:999-1012. [Crossref] [PubMed]
- Haroon Al Rasheed MR, Xu B. Molecular alterations in thyroid carcinoma. Surg Pathol Clin 2019;12:921-30. [Crossref] [PubMed]
- Fu D, Shi Y, Liu JB, et al. Targeting long non-coding RNA to therapeutically regulate gene expression in cancer. Mol Ther Nucleic Acids 2020;21:712-24. [Crossref] [PubMed]
- Niu L, Yang W, Duan L, et al. Biological implications and clinical potential of metastasis-related miRNA in colorectal cancer. Mol Ther Nucleic Acids 2020;23:42-54. [Crossref] [PubMed]
- Thomson DW, Dinger ME. Endogenous microRNA sponges: evidence and controversy. Nat Rev Genet 2016;17:272-83. [Crossref] [PubMed]
- Sanchez-Mejias A, Tay Y. Competing endogenous RNA networks: tying the essential knots for cancer biology and therapeutics. J Hematol Oncol 2015;8:30. [Crossref] [PubMed]
- Bayat Z, Ghaemi Z, Behmanesh M, et al. Hsa-miR-186-5p regulates TGFβ signaling pathway through expression suppression of SMAD6 and SMAD7 genes in colorectal cancer. Biol Chem 2021;402:469-80. [Crossref] [PubMed]
- Yao H, Yang Z, Lou Y, et al. miR-186 inhibits liver cancer stem cells expansion via targeting PTPN11. Front Oncol 2021;11:632976 [Crossref] [PubMed]
- Wan Q, Tang M, Sun SL, et al. SNHG3 promotes migration, invasion, and epithelial-mesenchymal transition of breast cancer cells through the miR-186-5p/ZEB1 axis. Am J Transl Res 2021;13:585-600. [PubMed]
- Ning Y, Bai Z. DSCAM-AS1 accelerates cell proliferation and migration in osteosarcoma through miR-186-5p/GPRC5A signaling. Cancer Biomark 2021;30:29-39. [Crossref] [PubMed]
- Ma Y, Zhang D, Wu H, et al. Circular RNA PRKCI silencing represses esophageal cancer progression and elevates cell radiosensitivity through regulating the miR-186-5p/PARP9 axis. Life Sci 2020;259:118168 [Crossref] [PubMed]
- Scheiblecker L, Kollmann K, Sexl V. CDK4/6 and MAPK-crosstalk as opportunity for cancer treatment. Pharmaceuticals (Basel) 2020;13:418. [Crossref] [PubMed]
- Wang PF, Qiu HY, He Y, et al. Cyclin-dependent kinase 4/6 inhibitors for cancer therapy: a patent review (2015 - 2019). Expert Opin Ther Pat 2020;30:795-805. [Crossref] [PubMed]
- Dall'Acqua A, Bartoletti M, Masoudi-Khoram N, et al. Inhibition of CDK4/6 as therapeutic approach for ovarian cancer patients: current evidences and future perspectives. Cancers (Basel) 2021;13:3035. [Crossref] [PubMed]
(English Language Editor: K. Brown)