Anatomic and physiological fundamentals for autologous breast reconstruction
Introduction
Breast reconstruction has become an important consideration for women after mastectomy. The goal is to re-create a breast mound that is naturally soft, durable, looks and feels like a normal breast, and can mature and naturally change with the patient over time. Women undergoing breast cancer surgery have become younger over the years and so reconstructive options ought to place greater emphasis on longevity. Documented trends include changes in patient demands and expectations; the disadvantages of implant reconstruction being better understood and the results through autologous reconstruction being more widely recognized (1,2).
Although the use of autologous tissue is less commonly performed compared to implant-based reconstruction it is still is seen as a preferable choice in circumstances and has gained increasing popularity over the years. Autologous tissue can behave very much like normal breast tissue, however the surgery is considered to be more complex and lengthy in comparison to prosthetic based reconstruction. However, the advancement of microsurgical and reconstructive techniques and incorporation of enhanced recovery protocols has significantly reduced the associated morbidity with autologous breast reconstruction.
The success of autologous tissue transfer is reliant on adequate blood supply and as we endeavour to tailor our reconstructive options through our flap choices and design. Autologous breast reconstruction has made substantial progress over the years and the evolution of refinements over the last 30 years has allowed flaps to be based on specific perforators. This revolutionary concept can preserve underlying muscle, reduce donor site morbidity and ability to tailor the flap to reconstruct exactly the tissues that are missing at the recipient site. The ultimate goal of breast reconstruction following mastectomy is to match optimal tissue replacement with minimal donor-site expenditure. In parallel surgeons will seek ways to ensure safe flap design and harvest while maintaining predictability and reliable tissue perfusion.
The mantra of Sir Harold Gillies that surgeons are “faced with a constant battle between vascular supply and beauty,” is a reflection of the challenges and goals of the reconstructive breast surgeon. The foundations of our vascular anatomical knowledge stems from pioneering works including that of Manchot, Salmon, Cormack and Lamberty, and Taylor and Palmer (3-5). Better understanding of the vascular anatomy and physiology of the cutaneous circulation of soft tissues, and that of patterns of blood flow from individual perforator has provided insight to advance perforator flap harvest and modifications in flap design.
The aim of this article is to review the principles of blood supply and flap design exemplified through common flaps used in autologous breast reconstructive surgery, to better understand approaches for safe flap harvest and transfer of well perfused tissue.
The perforasome theory
The angiosome theory defined by Taylor and Palmer in 1987 characterized the vasculature of the human body as organized into “Angiosomes” (5). Each angiosome refers to a block of tissue supplied by a source vessel and linked to each other via “choke vessels” in the subdermal plexus, named due to their relatively small calibre. The increasing use of perforator flaps advocated a critical need to better assess vascular architecture. Saint-Cyr et al. focused on the perforator itself and not the source vessel through a series of anatomical studies, to define individual vascular territories through 3D and 4D computed tomographic angiography (CTA). The “Perforasome” concept, coined by Saint-Cyr et al. in their original article, described how each cutaneous perforator had its own unique vascular arterial territory (6), and this has been referred to in other texts as a “perforator angiosome” (7) or “cutaneous angiosome” (8). Large filling pressures through a single dominant perforator can allow for large perforator flap harvest based on linking vessels that may connect multiple perforasomes to one another (9). Some key principles of the perforasome theory are summarized in Table 1.

Full table
First principle
Each perforasome is linked with adjacent perforasomes by means of two main mechanisms that include both direct and indirect linking vessels. Direct linking vessels are large vessels which allow flow from one perforator to the next and allow capture of adjacent perforasomes through an inter-perforator flow mechanism. Perforasomes are also linked to one another by indirect linking vessels or recurrent flow via the subdermal plexus (Figure 1). These vessels are similar to choke vessels described by Taylor et al. (10).
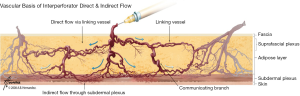
Second principle
Flap design and skin paddle orientation should be based on the direction of the linking vessels, which is axial in the extremities and perpendicular to the midline in the trunk. Orientation of the linking vessels corresponds to the orientation of maximal blood flow, and flap axis should ideally be designed with this consideration (Figure 2).
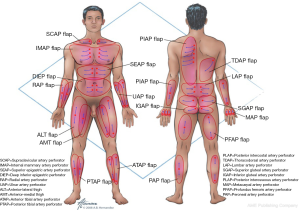
Third principle
Preferential vascular filling patterns occur within perforators of the same source artery first, followed by the perforators of other adjacent source arteries. The linking vessels then emanate from this main perforasome to perforasomes of adjacent vascular territories from other source arteries.
Fourth principle
Mass vascularity of a perforator found adjacent to an articulation is directed away from the same articulation. Whereas perforators found at a midpoint between two articulations or midpoint of the trunk has a multidirectional flow distribution. Therefore flap design should take into consideration the perforator location.
Successful autologous reconstruction relies on robust blood supply. We discuss the principles of vascular anatomy and flap design for autologous flaps for reconstructive breast surgery. A comprehensive understanding of the vascular anatomy is critical to evidence-based perforator selection and optimizing flap design.
Deep inferior epigastric perforator (DIEP) flap
The use of abdominal tissue for autologous breast reconstruction has been long and widely practiced, with similar characteristics to breast tissue and an aesthetic donor site scar. The DIEP flap had evolved from the traditional transverse rectus abdominis myocutaneous (TRAM) flap that was used for pedicled breast reconstruction based on the superior epigastric artery (11). However, the inferior epigastric artery plays the dominant role in abdominal tissue transfer in autologous reconstruction (12). Koshima and Soeda first described the DIEP flap in 1989 (13) and Allen and Treece popularized its use in breast reconstruction in 1994 (14). This flap has been studied extensively and is a safe reliable option in breast reconstruction with low morbidity (Figure 3).
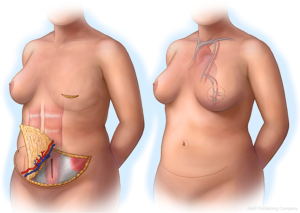
The artery arises from the external iliac artery and approaches the rectus muscle on its lateral edge and travels towards the arcuate line on its deep surface. At this level the main artery will form one of three typical branching patterns (15). The commonest branching pattern is a type II (57-89%), which is a simple bifurcation and perforators arising from the medial or lateral row of the DIEA. Type I vascular pattern involves a single inferior vessel (27-29%) and type III pattern (14-16%) is a trifurcating pattern above the arcuate line (16). Variations in anatomy include absent unilateral DIEA (17), duplicate systems and intra-abdominal origin of the DIEA (16). The perforators may take an intramuscular course, which can be short (most common), perpendicular or a long oblique course, or they may have a completely extra-muscular course. After exiting the rectus muscle, the perforators may directly pierce the anterior rectus sheath or travel a short distance in the sub-fascial plane before penetrating the anterior rectus sheath. In a systematic review by Ireton et al. [2014] the course of these perforators had described a considerable anatomical variation in that 20% up to 67% had a direct course on exiting the fascia, and around 33% and 50% may have a sub-fascial course between 0.5 up to 3 cm from the collated studies. Perforators that had a more direct course were found usually within 3 cm of the umbilicus, and those lower in the abdomen were more likely to have a longer sub-fascial course (16,18).
In the subcutaneous layer, lateral row perforators have a more oblique course through this layer, whilst the medial row perforators a more direct path. Once the perforators reach the subcutaneous and dermal layers, there is a considerable branching and anastomoses, with midline perforators presenting with a high degree of midline crossover, in contrast to the lateral row perforators that have little midline crossover of their vascular territories which has been demonstrated on cadaveric anatomical studies (7,19). Larger dominant perforators are typically seen in the medial row, and within 3-5 cm of the umbilicus, representing a “hot spot” of dominant perforators in DIEP flap harvest (Figure 4). There has been a considerable degree of anatomical, radiological and clinical studies using a variety of intraoperative technologies, to determine the perfusion patterns of perforators following DIEP flap harvest. In the harvest of the TRAM flap, where most of the DIEP perforators are included, it has been accepted that the perfusion of the flap integument occurs in zones. Four zones have been typically described for vascular perfusion of the lower abdominal wall, with sequential filling of each zone (11,20-22). The first zone universally represents the highest degree of perfusion found on the ipsilateral side of the DIEA perforator. The Hartrampf zones of perfusion (I to IV) are familiar to most plastic surgeons (Figure 5). The Hartrampf zones II and III were shown reversed by Holm et al. using intraoperative fluorescence imaging (22). There is contention in the literature of the characteristics of these zones and the application of these traditional zones to perforator flaps based on a single dominant perforator. It is recognized that lateral and medial row perforator have different vascular perfusion patterns through clinical, radiological and cadaveric anatomical studies (7,22-24).
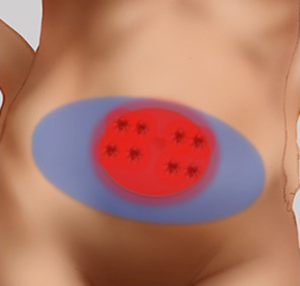
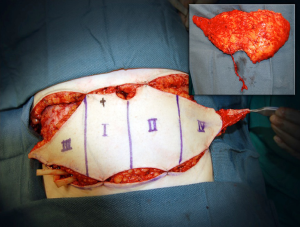
Medial row perforators generally are larger in calibre, with more extensive branching patterns and greater perfusion patterns compared to lateral row perforators (7,12,19,23,24). The medial row perforators can reliably perfuse across the midline and provide a robust vascularity to flaps raised on a single dominant perforator in the central two zones (19). The perforators are found to have a more direct course through the anterior rectus sheath (16) and to Scarpa’s fascia compared to lateral row perforators. The medial row perforasome can be classified using the traditional zone concepts and through anatomical studies have demonstrated perfusion patterns similar to Hartrampf zones of perfusion. In contrast, the lateral row perforasome is more representative of Holm’s zones of perfusion (23).
For unilateral reconstruction, the medial row perforators perfuse more medially and lateral perforators perfuse laterally, that is more concentrated to a hemi-abdomen (7), and they share a similar territory to the ipsilateral superficial inferior epigastric artery (SIEA) (23). The DIEP flap’s vascular territory could be potentially augmented with the inclusion of a second perforator. The lateral branches of the DIEA during flap harvest were frequently dominant and run a more rectilinear course which would permit an easier dissection (25). However, if the medial row perforators were dominant, it would be recommended to harvest a flap based on a dominant medial row perforator, particularly if a larger flap is being required (as an alterative to muscle-sparing TRAM flap). Although in the past it was advocated that if a flap was reconstructed with only a hemi-abdominal flap, a lateral row perforator DIEA or SIEA could be considered. However, perfusion territories can vary between medial and lateral row, the decision for perforator choice should be based on the largest and dominant perforator in the hemi-abdomen, regardless of row.
In the hemi-abdomen the medial and lateral row are connected by direct linking vessels and indirect linking vessels via the subdermal plexus (Figure 6). For medial row perforators, there are large linking vessels, which connect with the lateral row and additional intra-row perforators; linkage with the contralateral medial row perforators across the midline is obtained via the subdermal plexus. These linking vessels are similar to the choke vessels described by Taylor. The use of preoperative CTA can be used preoperatively, to review the presence of dominant perforators and linking vessels, and the course of the dominant perforators to plan the dissection and flap harvest (26,27). The addition of intraoperative use of indocyanine green laser fluorescence angiography has provided a useful tool for an early assessment of flap perfusion and micro-anastomotic flow (Figure 7).
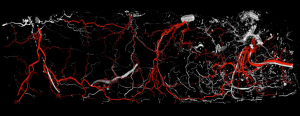
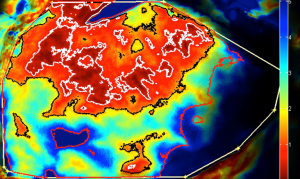
A newer model proposed for perforator perfusion is centred on the dominant perforator and perfusion falls sequentially between adjacent perforasomes (7,9). For example, zone I supplied by an ipsilateral medial row DIEA perforator, the immediate adjacent perforasome zone II is captured by the ipsilateral lateral row DIEA and contralateral medial row DIEA; zone III, refers to the second captured perforasome of the contralateral lateral row and ipsilateral SIEA territory, and Zone IV refers to the contralateral SIEA territory (6,9) (Figure 8).
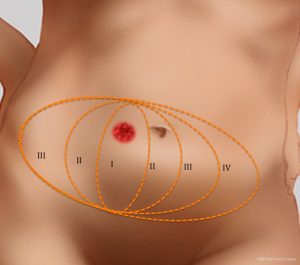
Linking vessels in the trunk are commonly directed perpendicular to the midline and follow an oblique transverse direction, parallel to the cutaneous dermatomes. Flow from these perforator angiosomes can be multidirectional and cross the midline in many cases, but preferential flow is normally directed away from the midline to maintain adequate blood supply to adjacent regions, which are populated with fewer perforators (Figure 2).
Venous outflow can be the limiting factor in DIEP reconstruction leading to flap failure (28), but although venous compromise is multifactorial, the physiology of venous outflow is still poorly understood. The incidence of venous congestion in DIEP flaps has ranged from 3 to 27 percent in the literature, and the higher rates are usually recognized intra-operatively (Figure 9A) (29). Proposed mechanisms include the perforator being too small, absence of midline crossover veins except for indirect linking vessels at the subdermal plexus level found in up to 36% of cases in anatomical studies (30), connections between the superficial and deep systems (28,31). Although arterial inflow from DIEA is often reliable the DIEV may be inadequate and therefore augmentation of venous drainage may be required. A variety of approaches have been adopted to supercharge or augment the venous drainage of the DIEP flap. These approaches generally incorporate the use of a “lifeboat” option during DIEP flap harvest, including dissecting out the superficial inferior epigastric vein (SIEV), a medial branch of the SIEV (MSIEV) or additional perforator (Figure 9B). Additional venous anastomoses include using both venae comitantes, connection of the SIEV intrinsically within the flap (to a deep perforator venae comitante) or extrinsically (e.g., anterograde or retrograde to the internal mammary vein) (29).
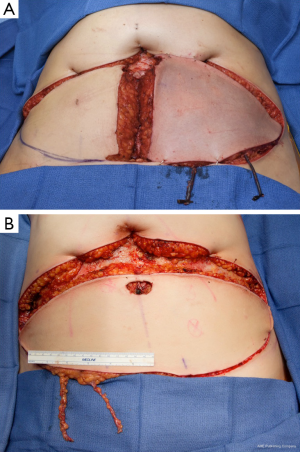
The diameters of the DIEA, DIEV, perforator veins and arteries have been correlated but independent of the SIEV in cadaveric studies (31-33). Cadaveric injection studies by Carramenha e Costa et al. first described that venous drainage of abdominal wall was dominated by the superficial system, but this still warrants further investigation (32). In anatomical studies carried out by Schaverien et al. [2008] (24), superficial and deep venous drainage systems were connected by the venae comitantes of the perforators of the DIEA and injection studies of either revealed similar venous filling patterns, including those of adjacent venae comitantes. Adjacent superficial epigastric vein filling patterns crossed the midline at the level of the subdermal plexus (30).
Dominant perforators of the deep system concentrate around the periumbilical area (32) and flap design should include perforating veins at the level of the umbilicus. However, inadequate communication between the chosen perforator venae comitantes and the SIEV system can be responsible for diffuse venous congestion seen in some DIEP flaps (30). Schaverien et al. [2010] reviewed venous anatomy on preoperative magnetic resonance angiography (MRA) and identified that venae comitantes with direct venous connections to the SIEV were significantly more likely to be found in the medial row, demonstrating that medial row perforators may provide more adequate venous drainage to a DIEP flap (30). It is important to highlight that the sensitivity of detecting small communicating vessels between the deep and superficial systems can be limited in CTA and MRA for very small vessels. However, Rozen et al. [2012] have described the presence macrovascular shunts identified on preoperative CTA (a direct communicating vessel between a DIEA and SIEV territory), which warrants further investigation (34).
In addition to flap harvest for breast reconstruction, we have adopted a conservative approach to the dissection of the abdominoplasty flap for donor site closure. This technique involves a limited lateral dissection to preserve lateral perforators to the abdominoplasty skin flap, and a central dissection that proceeds to the xiphoid process (Figure 10) (35,36).
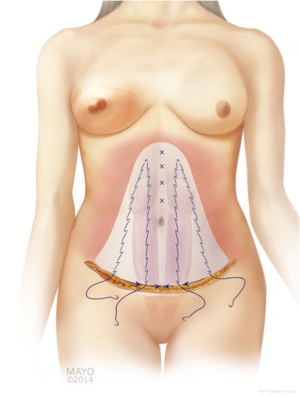
A better understanding of vascular anatomy and zones of perfusion of perforators in DIEP flap harvest for breast reconstruction will help to improve the predictability and reliability of flap harvest, and decrease flap-related complications, such fat necrosis, and partial flap loss. Linking vessels are a key component to understand the full potential of vascular territories of individual perforators and the overlap with vascular territories of other source arteries. Anatomical, radiological and intraoperative imaging studies have provided fundamental knowledge base to our understanding of vascular anatomy, although there are many areas that warrant going research for DIEP breast reconstruction.
Key points of DIEP anatomy for flap design
- Flaps should be raised on the largest and dominant perforator regardless of row. Dominant perforators are usually found in a “hot spot” within 3-5 cm of the umbilicus;
- Lateral perforators commonly have a more rectilinear course and perfusion is concentrated more to the hemi-abdomen;
- Medial row perforators exhibit a greater degree of branching and larger calibre direct and indirect linking vessels via the subdermal plexus, which provides more robust vascularity across the midline, in contrast to lateral row perforators;
- When a large amount of tissue is required, either a dominant medial row perforator or muscle-sparing TRAM should be preferentially chosen for flap harvest;
- The use of preoperative CTA can identify the largest perforators in the lower abdomen, their course, and the presence of large linking vessels intra-row and inter-row, which can provide some inference to overall flap vascularity;
- Venous systems can be assessed to a certain degree with preoperative imaging and connections between the superficial and deep systems. However, lifeboat options should be considered and incorporated in the flap harvest e.g., inclusion of the SIEV routinely within the flap;
- Intraoperative imaging including fluorescence laser angiography is gaining popularity in reconstructive surgery as an intraoperative adjunct to assess flap perfusion and venous congestion for early identification of perfusion changes.
Transverse myocutaneous upper gracilis flap
The transverse myocutaneous gracilis, also known as the transverse upper gracilis (TUG) flap, has been utilized in breast reconstruction since its anatomical description in 1992 (37-42). It can be used in bilateral reconstruction or stacked for a unilateral reconstruction. A perceived disadvantage with the flap is the low volume of harvested tissue compared with abdominal and gluteal flaps, which has traditionally limited this technique to small and to mid-size breasts. Medial thigh tissue correlates well with body mass index, and therefore a good option if abdominal or alternative donor sites are not available. Harvesting tissue from a more posterior location is a modification we have adopted to take opportunity of the bulkier posterior thigh tissue (39-41) allowing further recruitment of tissue into the flap. We discuss the basic anatomy and these modifications described as the extended TUG flap. Saint-Cyr et al. have shown this surgical approach to be appropriate to gain size without increased morbidity (43).
The gracilis is a flat type II (Mathes and Nahai) muscle flap of the medial thigh and in the presence of adductor longus and magnus it is expendable. The dominant pedicle arises from the ascending branch of the medial circumflex artery or directly from the profunda femoris and enters the muscle belly on its deep surface in the upper third, at 9-12 cm inferior to the pubic tubercle. Usually two or three vascular pedicles enter the muscle, the most proximal usually being the dominant pedicle. The dominant pedicle can provide up to 6-7 cm in the length and on entering the muscle it divides into an ascending, descending, transverse branches running in parallel with the longitudinal muscle fibres and commonly anastomosing with the second vascular pedicle. The dominant pedicle provides musculocutaneous perforators to the overlying skin in the proximal third of the muscle and direct fasciocutaneous vessels from the medial circumflex artery, or superficial femoral artery in the distal portions of the muscle.
The skin paddle was traditionally designed longitudinally with variable distal perfusion, however Yousif et al. showed that perforators had a tendency to travel in a horizontal direction with perforators posterior to the gracilis muscle and this led to re-design of the skin paddle (37). The potential skin territory is thought to extend from the rectus femoris to the biceps femoris and selective angiographic studies of the dominant pedicles have demonstrated vascular cutaneous territories of up to 400 cm2, with the perfusion territory extending posteriorly to the gracilis muscle (44).
The skin paddle superior border is roughly 1-2 cm below the upper thigh crease concealing the donor TUG flap specifically, skin paddles can be raised up to approximately 16.5 cm × 11 cm or more, but dependent on skin laxity. The maximal anterior extension of the horizontal skin paddle is approximately 1-2 cm anterior to the lateral edge of adductor longus to avoid disruption the lymphatic basic or create a noticeable scar. Variations of the skin paddle design include combination with a vertical skin paddle, to create a tri-lobed (Figure 11), “L” shaped skin paddle, or “S” shaped design (Figure 12). When designing the tri-lobed pattern, it is important to keep the base of each lobe relatively broad which would result in an oval shape at the centre of the flap (Figure 11A). When wanting to incorporate horizontal and transverse laxity, the senior author would prefer the “S” design to avoid the problematic T-junction at the donor site resultant from the tri-lobed design. The vertical incision should be placed at the anterior border of the gracilis and stopped at mid-thigh level to avoid the unreliable perfusion zone of the distal gracilis territory. Fattah et al. have described undermining of the inferior skin incision in a bevelled fashion to recruit subcutaneous fat over the gracilis muscle and posteriorly to add flap volume, but it is restricted in the widest portion of the flap to avoid wound closure with undue tension (39).
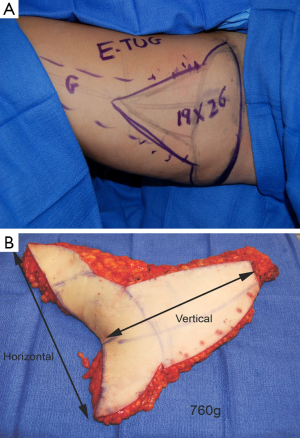
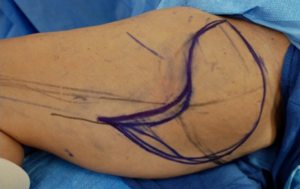
A dominant blood supply of the extended TUG flap is concentrated posteriorly (Figure 13). Therefore the posterior extension of the flap is carried out midway between the medial mid-axial line and posterior thigh midline. Any extension beyond this point may increase the risk of fat necrosis, damage to posterior cutaneous nerves, and flap-related complications. The extended transverse upper gracilis flap has consistent vascular reliability, with enough tissue for reconstruction of small to moderate sized breasts. In a retrospective study of 12 extended transverse gracilis flaps, the average flap weight was 386 grams, yielding up to 750 grams on the large size (7). The approaches described for skin paddle modifications, incorporate knowledge of potential perfusion territories, and methods to reliably maximize volume without additional morbidity (Figure 14A,B).
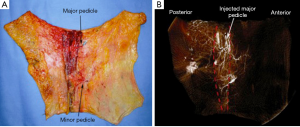
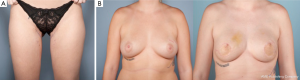
Key points of vascular anatomy for TUG flap design
- Reconstruct small to medium breasts;
- The dominant blood supply extends posteriorly and over the proximal medial thigh (distal skin perfusion is unreliable) which must be considered when designing the skin paddle;
- The extended TUG flap can optimize recruitment of posterior and medial thigh subcutaneous tissue;
- Subcutaneous tissue recruitment should be concentrated over the gracilis muscle and posteriorly only;
- Limit anterior extension of the skin paddle, as this less reliably perfused and limited anterior dissection will.
Thoracodorsal artery perforator (TDAP) flap
The thoracodorsal artery perforator (TDAP) flap was first described by Angrigiani and colleagues (45) in which the cutaneous island of latissimus dorsi (LD) musculocutaneous flap was raised on one perforator without the LD muscle included. This flap is not as commonly used in autologous breast reconstruction thought to have anatomic consistencies of the perforators compared to other autologous perforator flap options. However the donor site is aesthetically acceptable and permits the preservation of the underlying LD muscle without seroma formation.
The perforating branching of the TDAP originate from the descending and transverse branches of the thoracodorsal artery, with the most dominant reliable perforators arising from the descending branch which courses along a parallel line 2 cm from the anterior edge of the latissimus muscle (46). There are around 2-3 cutaneous perforators along this course, with the proximal perforator, which is usually the largest, found approximately 8 cm below the posterior axillary fold or at the angle of the inferior angle of the scapula. This proximal perforator is usually close to the hilum of the thoracodorsal artery and nerves. In a study by Schaverien et al. [2010] the incidence of distal perforators along the course of the descending branch decreased in 15 anatomical cadaveric dissections (47). In this study it was also noted that in 53% of the dissections a direct extramuscular branch from the thoracodorsal artery was observed coursing over the lateral edge of the muscle and arising as a septocutaneous perforator. In this study, CTA injection studies demonstrated the perfusion of these flaps occurred through direct and indirect linking vessels in a similar manner to DIEP flaps.
Muscle-sparing latissimus dorsi (MSLD) flap
Following on from the previous description of the TDAP flap, the MSLD flap can be used for pedicled breast reconstruction without or without combined tissue expander. In this form of autologous breast reconstruction, only the anterior portion of the LD muscle is harvested with the skin paddle. The cutaneous skin paddle of this flap and preoperative markings are based on the anatomy of perforating branches of the descending branch of the thoracodorsal artery previously described (Figure 15). Advantages over its thoracodorsal perforator-based counterpart includes: simple technical dissection, versatility in flap design irrespective of adequately sized perforator location, and better neurovascular pedicle protection due to the small muscle cuff retained (48,49). In the presence of a previously irradiated bed, our preferential reconstruction would be an extended LD myocutaneous flap incorporating the entire muscle.
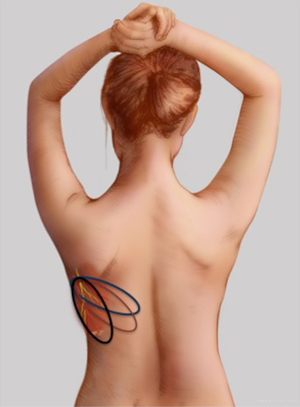
The thoracodorsal artery provides the dominant blood supply to the LD muscle in addition to segmental perforating branches from the intercostal and lumbar arteries (Mathes and Nahai type V circulatory pattern). The thoracodorsal artery pierces the LD muscle 10-11 cm distal to the origin of its insertion (50) and bifurcates into the transverse and descending branches, with significant overlap of vascular territories via cross-linking vessels (51,52). The average distance from the axillary artery, the subscapular artery and posterior axillary fold to the bifurcation is approximately 8, 4, and 5 cm respectively (51). The mean length of the descending branch is around 15 cm (48). The descending branch musculocutaneous perfusion territory covers around 87 percent relative to the thoracodorsal artery perfusion (51). The area of greater perforator density can be found between 9 to 15 cm from the posterior axillary fold and within 4 cm from the lateral edge of the latissimus muscle (47). The descending branch of the thoracodorsal artery can then be identified coursing along the under surface of the muscle. A 3-4 cm pedicle width of muscle from the anterior border is usually harvested.
Unlike the thoracodorsal artery perforator flap, the skin paddle for the descending branch muscle-sparing LD flap is not dependant on a specific perforator. The skin paddle location is designed irrespective of perforator location and can be positioned at any level along the axis of the descending branch of the thoracodorsal artery. The skin paddle, if required, is oriented transversely or slightly oblique along one of the natural adipose tissue rolls of the lower back to maximize flap dimension, place the donor scar along a natural skin crease (Figure 16). Skin paddle perfusion can be optimized by centring it over the LD muscle cuff harvested along the descending branch, and consideration of perforator density in the area described above (47,51). The most lateral edge of the skin paddle should be 1-2 cm anterior to the muscle border to optimize perforators captured and therefore flap perfusion (Figure 17A-C) (53). Positioning the skin paddle lower in the back will provide a longer pedicle with a greater amount of freedom in the arc of rotation. The skin paddle can be raised off the muscle outside the limits of the planned muscle pedicle width. A large arc of rotation is made possible by two flap pivot points that are located at the flap muscle juncture and at the proximal bifurcation point (Figure 18) (53).
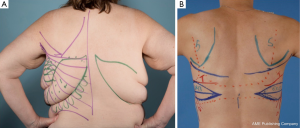
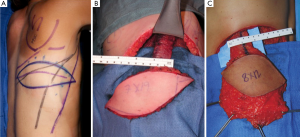
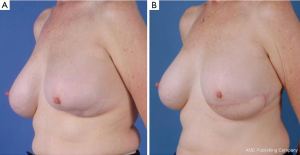
Key points of vascular anatomy for MSLD flap design
- Based on dominant large perforators arising from the descending branch of the thoracodorsal artery, with usually 3-4 cutaneous perforators identified along its course;
- The largest perforators are usually found proximally at the level of the inframammary fold or inferior angle of the scapula;
- The skin paddle is not designed over a specific perforator unlike the TDAP flap;
- A 4-cm anterior muscle pedicle is raised with a skin paddle, which can be dissected off the latissimus muscle, except over the planned muscle pedicle. This creates a two point pivot intrinsic to the flap and adds further versatility for inset.
Profunda artery perforator (PAP) flaps
The PAP flap is a relatively newer fasciocutaneous option used in autologous breast reconstruction based on musculocutaneous perforators of the posteromedial thigh. The donor site can be conspicuous and scar well-hidden.
The medial and posterior aspects of the thigh have abundant skin and excess subcutaneous tissue. Angrigiani et al. [2001] described the adductor flap based on the medial and posterior aspects of the thigh based on the musculocutaneous perforator from the first medial branch of the profunda femoris artery (54).The largest part of the posterior skin territory is supplied by the profunda femoris artery, mostly by the first and second PAPs (55). Smaller areas supplied by the adjacent vascular territories from the superficial femoral artery and inferior gluteal artery (56,57).
The proximal cutaneous perforator of the adductor magnus muscle is a major contributor to the vascular perfusion of the medial and posterior thigh skin and one of the largest musculocutaneous perforators of the body (58,59). This flap has been used as a free transfer with a transverse skin paddle for breast reconstruction when a gracilis flap or cutaneous branch of the medial circumflex artery artery was not appropriate (Figure 19) (60). Cormack and Lamberty identified a consistent proximal cutaneous perforator of the adductor magnus which is consistently located 2 cm posterior to the posterior border of the gracilis and 8 cm inferior to the groin crease. The second medial branch of the profunda femoris artery originates at approximately the same level as the second lateral perforating branch and distributes perforators to the semimembranosus and semitendinosus, and pierces the mass of the adductor muscle (61). In previous study by Saad et al. [2012] the dominant perforator for this flap was consistently found within 5 cm of the inferior gluteal crease and on average 6.2 cm form the posterior midline (60) and average pedicle length of 10.6 cm. Skin paddles are traditionally orientated transversely for breast reconstruction, with a maximal width of 8 cm to ensure direct closure however, as with the TUG flap, variations on design can be adapted with consideration of the tissue laxity. In our cadaveric study, the dominant proximal perforator was musculocutaneous 70% of the time, however, sometimes it did arise between the adductor magnus and semitendinosus. The mean pedicle length harvested was 10.4 cm, mean diameter of the vessels of 3.5 mm at the origin and average perforator injection territory was 265 cm2. The largest concentration of perforators was located in the upper medial posterior thigh region, which is relevant to autologous breast reconstruction. Large linking vessels were seen traversing from medial to lateral within the upper posterior thigh, and perforators that arose and travelled more obliquely towards the posterior midline of the thigh, had a bidirectional axiality of flow (Figure 20).
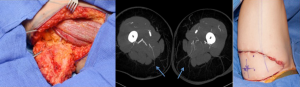
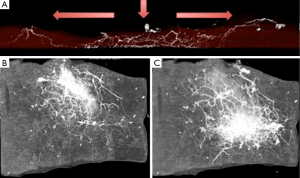
Superior (SGAP) and inferior gluteal artery perforator (IGAP) flaps
The superior and inferior gluteal myocutaneous flaps have been described in breast reconstruction in 1975 and 1978 respectively (62,63). Koshima et al. described its use as a perforator flap, however its use in breast reconstruction as a SGAP flap was described by Allen and Tucker in 1993 (64,65). The IGAP flap is the first line alternative for autologous breast reconstruction for some surgeons when the lower abdominal donor site is of insufficient volume. As perforator flaps, the advantages of these flaps include a hidden scar and low donor-site tissue, good volume of tissue can usually be ascertained which has a similar feel to breast tissue (Figure 21). There may be an abundance of donor tissue in this area even in slim patients.
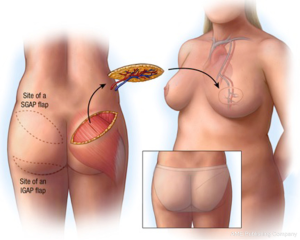
The superior and inferior gluteal arteries are terminal branches of the internal iliac artery, exiting the greater sciatic foramen superior and inferiorly to the piriformis muscle. The inferior pedicle is accompanied by the posterior cutaneous nerve of the thigh and greater sciatic nerve. The SGAP cutaneous territory will have around three perforators, whilst the IGAP territory has 2-4 perforators on average. The pedicle length of the IGAP is usually longer at around 7-10 cm, as it courses more obliquely through the gluteus maximus muscle, compared to the 3-8 cm pedicle length of the SGAP. The vascular territory of an individual perforator can reliably perfuse the whole region of the flap, and perfusion extends to adjacent perforator territories through direct linking vessels and recurrent flow via the subdermal plexus of the flap (66).
Conclusions
Blood supply is critical to successful autologous breast reconstruction. A fundamental appreciation of the vascular anatomy and integrity of flap designs used in breast reconstruction provides the foundation knowledge to ensure safe, more predictable flap harvest and provides scope for further modifications whilst maintaining a robust blood supply.
Acknowledgments
Disclosure: Dr. Saint-Cyr is a Consultant for Allergan, Mentor and Lifecell. The other author declares no conflict of interest.
References
- Levine SM, Lester ME, Fontenot B, et al. Perforator flap breast reconstruction after unsatisfactory implant reconstruction. Ann Plast Surg 2011;66:513-7. [PubMed]
- Hamdi M, Andrades P, Thiessen F, et al. Is a second free flap still an option in a failed free flap breast reconstruction? Plast Reconstr Surg 2010;126:375-84. [PubMed]
- Manchot C. eds. The cutaneous arteries of the human body. New York: Springer-Verlag, 1983.
- Cormack GC, Lamberty BG. Fasciocutaneous vessels. Their distribution on the trunk and limbs, and their clinical application in tissue transfer. Anat Clin 1984;6:121-31. [PubMed]
- Taylor GI, Palmer JH. The vascular territories (angiosomes) of the body: experimental study and clinical applications. Br J Plast Surg 1987;40:113-41. [PubMed]
- Saint-Cyr M, Wong C, Schaverien M, et al. The perforasome theory: vascular anatomy and clinical implications. Plast Reconstr Surg 2009;124:1529-44. [PubMed]
- Rozen WM, Ashton MW, Le Roux CM, et al. The perforator angiosome: a new concept in the design of deep inferior epigastric artery perforator flaps for breast reconstruction. Microsurgery 2010;30:1-7. [PubMed]
- Taylor GI, Corlett RJ, Dhar SC, et al. The anatomical (angiosome) and clinical territories of cutaneous perforating arteries: development of the concept and designing safe flaps. Plast Reconstr Surg 2011;127:1447-59. [PubMed]
- Saint-Cyr M. Assessing perforator architecture. Clin Plast Surg 2011;38:175-202. [PubMed]
- Taylor GI, Caddy CM, Watterson PA, et al. The venous territories (venosomes) of the human body: experimental study and clinical implications. Plast Reconstr Surg 1990;86:185-213. [PubMed]
- Hartrampf CR, Scheflan M, Black PW. Breast reconstruction with a transverse abdominal island flap. Plast Reconstr Surg 1982;69:216-25. [PubMed]
- Wong C, Saint-Cyr M, Arbique G, et al. Three- and four-dimensional computed tomography angiographic studies of commonly used abdominal flaps in breast reconstruction. Plast Reconstr Surg 2009;124:18-27. [PubMed]
- Koshima I, Soeda S. Inferior epigastric artery skin flaps without rectus abdominis muscle. Br J Plast Surg 1989;42:645-8. [PubMed]
- Allen RJ, Treece P. Deep inferior epigastric perforator flap for breast reconstruction. Ann Plast Surg 1994;32:32-8. [PubMed]
- Moon HK, Taylor GI. The vascular anatomy of rectus abdominis musculocutaneous flaps based on the deep superior epigastric system. Plast Reconstr Surg 1988;82:815-32. [PubMed]
- Ireton JE, Lakhiani C, Saint-Cyr M. Vascular anatomy of the deep inferior epigastric artery perforator flap: a systematic review. Plast Reconstr Surg 2014;134:810e-821e. [PubMed]
- Whitaker IS, Rozen WM, Smit JM, et al. Peritoneo-cutaneous perforators in deep inferior epigastric perforator flaps: a cadaveric dissection and computed tomographic angiography study. Microsurgery 2009;29:124-7. [PubMed]
- Kikuchi N, Murakami G, Kashiwa H, et al. Morphometrical study of the arterial perforators of the deep inferior epigastric perforator flap. Surg Radiol Anat 2001;23:375-81. [PubMed]
- Bailey SH, Saint-Cyr M, Wong C, et al. The single dominant medial row perforator DIEP flap in breast reconstruction: three-dimensional perforasome and clinical results. Plast Reconstr Surg 2010;126:739-51. [PubMed]
- Scheflan M, Dinner MI. The transverse abdominal island flap: part I. Indications, contraindications, results, and complications. Ann Plast Surg 1983;10:24-35. [PubMed]
- Scheflan M, Dinner MI. The transverse abdominal island flap: Part II. Surgical technique. Ann Plast Surg 1983;10:120-9. [PubMed]
- Holm C, Mayr M, Höfter E, et al. Perfusion zones of the DIEP flap revisited: a clinical study. Plast Reconstr Surg 2006;117:37-43. [PubMed]
- Wong C, Saint-Cyr M, Mojallal A, et al. Perforasomes of the DIEP flap: vascular anatomy of the lateral versus medial row perforators and clinical implications. Plast Reconstr Surg 2010;125:772-82. [PubMed]
- Schaverien M, Saint-Cyr M, Arbique G, et al. Arterial and venous anatomies of the deep inferior epigastric perforator and superficial inferior epigastric artery flaps. Plast Reconstr Surg 2008;121:1909-19. [PubMed]
- Blondeel PN. One hundred free DIEP flap breast reconstructions: a personal experience. Br J Plast Surg 1999;52:104-11. [PubMed]
- Alonso-Burgos A, García-Tutor E, Bastarrika G, et al. Preoperative planning of deep inferior epigastric artery perforator flap reconstruction with multislice-CT angiography: imaging findings and initial experience. J Plast Reconstr Aesthet Surg 2006;59:585-93. [PubMed]
- Masia J, Clavero JA, Larrañaga JR, et al. Multidetector-row computed tomography in the planning of abdominal perforator flaps. J Plast Reconstr Aesthet Surg 2006;59:594-9. [PubMed]
- Blondeel PN, Arnstein M, Verstraete K, et al. Venous congestion and blood flow in free transverse rectus abdominis myocutaneous and deep inferior epigastric perforator flaps. Plast Reconstr Surg 2000;106:1295-9. [PubMed]
- Galanis C, Nguyen P, Koh J, et al. Microvascular lifeboats: a stepwise approach to intraoperative venous congestion in DIEP flap breast reconstruction. Plast Reconstr Surg 2014;134:20-7. [PubMed]
- Schaverien MV, Ludman CN, Neil-Dwyer J, et al. Relationship between venous congestion and intraflap venous anatomy in DIEP flaps using contrast-enhanced magnetic resonance angiography. Plast Reconstr Surg 2010;126:385-92. [PubMed]
- Rozen WM, Pan WR, Le Roux CM, et al. The venous anatomy of the anterior abdominal wall: an anatomical and clinical study. Plast Reconstr Surg 2009;124:848-53. [PubMed]
- Carramenha e Costa MA, Carriquiry C, Vasconez LO, et al. An anatomic study of the venous drainage of the transverse rectus abdominis musculocutaneous flap. Plast Reconstr Surg 1987;79:208-17. [PubMed]
- Figus A, Wade RG, Gorton L, et al. Venous perforators in DIEAP flaps: an observational anatomical study using duplex ultrasonography. J Plast Reconstr Aesthet Surg 2012;65:1051-9. [PubMed]
- Rozen WM, Ashton MW. The venous anatomy of the abdominal wall for Deep Inferior Epigastric Artery (DIEP) flaps in breast reconstruction. Gland Surg 2012;1:92-110. [PubMed]
- Nagarkar P, Lakhiani C, Cheng A, et al. No-drain DIEP flap donor site closure using barbed progressive tension sutures. Plast Reconstruct Surg GO 2015. (In press).
- Mohan AT, Rammos CK, Gaba P, et al. Modified aesthetic abdominoplasty approach in perforator free-flap breast reconstruction: Impact of drain free donor site on patient outcomes. J Plast Reconstr Aesthet Surg 2015. [Epub ahead of print]. [PubMed]
- Yousif NJ, Matloub HS, Kolachalam R, et al. The transverse gracilis musculocutaneous flap. Ann Plast Surg 1992;29:482-90. [PubMed]
- Arnez ZM, Pogorelec D, Planinsek F, et al. Breast reconstruction by the free transverse gracilis (TUG) flap. Br J Plast Surg 2004;57:20-6. [PubMed]
- Fattah A, Figus A, Mathur B, et al. The transverse myocutaneous gracilis flap: technical refinements. J Plast Reconstr Aesthet Surg 2010;63:305-13. [PubMed]
- Schoeller T, Huemer GM, Wechselberger G. The transverse musculocutaneous gracilis flap for breast reconstruction: guidelines for flap and patient selection. Plast Reconstr Surg 2008;122:29-38. [PubMed]
- Schoeller T, Wechselberger G. Breast reconstruction by the free transverse gracilis (TUG) flap. Br J Plast Surg 2004;57:481-2. [PubMed]
- Wechselberger G, Schoeller T. The transverse myocutaneous gracilis free flap: a valuable tissue source in autologous breast reconstruction. Plast Reconstr Surg 2004;114:69-73. [PubMed]
- Saint-Cyr M, Wong C, Oni G, et al. Modifications to extend the transverse upper gracilis flap in breast reconstruction: clinical series and results. Plast Reconstr Surg 2012;129:24e-36e. [PubMed]
- Whitaker IS, Karavias M, Shayan R, et al. The gracilis myocutaneous free flap: a quantitative analysis of the fasciocutaneous blood supply and implications for autologous breast reconstruction. PLoS One. 2012;7:e36367. [PubMed]
- Angrigiani C, Grilli D, Siebert J. Latissimus dorsi musculocutaneous flap without muscle. Plast Reconstr Surg 1995;96:1608-14. [PubMed]
- Thomas BP, Geddes CR, Tang M, et al. The vascular basis of the thoracodorsal artery perforator flap. Plast Reconstr Surg 2005;116:818-22. [PubMed]
- Schaverien M, Wong C, Bailey S, et al. Thoracodorsal artery perforator flap and Latissimus dorsi myocutaneous flap--anatomical study of the constant skin paddle perforator locations. J Plast Reconstr Aesthet Surg 2010;63:2123-7. [PubMed]
- Saint-Cyr M, Nagarkar P, Schaverien M, et al. The pedicled descending branch muscle-sparing latissimus dorsi flap for breast reconstruction. Plast Reconstr Surg 2009;123:13-24. [PubMed]
- Hamdi M, Van Landuyt K, Monstrey S, et al. Pedicled perforator flaps in breast reconstruction: a new concept. Br J Plast Surg 2004;57:531-9. [PubMed]
- Bartlett SP, May JW Jr, Yaremchuk MJ. The latissimus dorsi muscle: a fresh cadaver study of the primary neurovascular pedicle. Plast Reconstr Surg 1981;67:631-6. [PubMed]
- Colohan S, Wong C, Lakhiani C, et al. The free descending branch muscle-sparing latissimus dorsi flap: vascular anatomy and clinical applications. Plast Reconstr Surg 2012;130:776e-787e. [PubMed]
- Tobin GR, Schusterman M, Peterson GH, et al. The intramuscular neurovascular anatomy of the latissimus dorsi muscle: the basis for splitting the flap. Plast Reconstr Surg 1981;67:637-41. [PubMed]
- Wong C, Saint-Cyr M. The pedicled descending branch muscle-sparing latissimus dorsi flap for trunk and upper extremity reconstruction. J Plast Reconstr Aesthet Surg 2010;63:623-32. [PubMed]
- Angrigiani C, Grilli D, Thorne CH. The adductor flap: a new method for transferring posterior and medial thigh skin. Plast Reconstr Surg 2001;107:1725-31. [PubMed]
- Rubin JA, Whetzel TP, Stevenson TR. The posterior thigh fasciocutaneous flap: vascular anatomy and clinical application. Plast Reconstr Surg 1995;95:1228-39. [PubMed]
- Cormack GC, Lamberty BG. The blood supply of thigh skin. Plast Reconstr Surg 1985;75:342-54. [PubMed]
- Ahmadzadeh R, Bergeron L, Tang M, et al. The posterior thigh perforator flap or profunda femoris artery perforator flap. Plast Reconstr Surg 2007;119:194-200; discussion 201-2. [PubMed]
- Allen RJ, Haddock NT, Ahn CY, et al. Breast reconstruction with the profunda artery perforator flap. Plast Reconstr Surg 2012;129:16e-23e. [PubMed]
- Salmon M. eds. Les arteres de la peau. Paris: Masson, 1936.
- Saad A, Sadeghi A, Allen RJ. The anatomic basis of the profunda femoris artery perforator flap: a new option for autologous breast reconstruction--a cadaveric and computer tomography angiogram study. J Reconstr Microsurg 2012;28:381-6. [PubMed]
- Cormack GC, Lamberty BG. eds. The arterial anatomy of skin flaps. 1st ed. Edinburgh: Churchill Livingstone, 1989.
- Fujino T, Harasina T, Aoyagi F. Reconstruction for aplasia of the breast and pectoral region by microvascular transfer of a free flap from the buttock. Plast Reconstr Surg 1975;56:178-81. [PubMed]
- Le-Quang C. Secondary microsurgical reconstruction of the breast and free inferior gluteal flap. Ann Chir Plast Esthet 1992;37:723-41. [PubMed]
- Koshima I, Moriguchi T, Soeda S, et al. The gluteal perforator-based flap for repair of sacral pressure sores. Plast Reconstr Surg 1993;91:678-83. [PubMed]
- Allen RJ, Tucker C Jr. Superior gluteal artery perforator free flap for breast reconstruction. Plast Reconstr Surg 1995;95:1207-12. [PubMed]
- Saint-Cyr M, Schaverien M, Arbique G, et al. Three- and four-dimensional computed tomographic angiography and venography for the investigation of the vascular anatomy and perfusion of perforator flaps. Plast Reconstr Surg 2008;121:772-80. [PubMed]