Recovery of laryngeal function after intraoperative injury to the recurrent laryngeal nerve
Recurrent laryngeal nerve (RLN) injury and voice alteration after thyroid surgery
Thyroid and parathyroid surgery is associated with a risk of traumatic injury to the superior and inferior RLN. Injury to the RLN results in acute paralysis of the vocal fold on the affected side, which leads to dysphonia, dysphagia, and aspiration problems. The clinical signs may vary, however, depending on the position of the paralyzed vocal fold relative to the midline and the degree of glottic insufficiency. A significant number of patients may even present as asymptomatic, and vocal fold mobility needs to be examined before and after surgery to detect an iatrogenic injury to the RLN (1-4). The reported risk for RLN injury after thyroid/parathyroid surgery varies from center to center. In the literature, injury rates of up to 38% can be found (5). It is difficult to compare these numbers, since the rate of detected RLN injury is dependent on how close to surgery the laryngoscopic examination is performed. In a large, retrospective study of patients that underwent total thyroidectomy due to malignant disease, the risk of postoperative vocal fold paresis was found to be 9.5% (6), of which 22% became permanent with resulting demand for secondary surgical intervention of the paralyzed vocal fold.
Subjective voice deficits are common after thyroid surgery (7). Subjective and objective voice alterations (8,9) are common after total thyroidectomy and most of them are independent of injury to the RLN or the superior laryngeal nerve (SLN) (10-12). These voice changes are believed to be caused by temporary disturbances in the laryngeal mechanical framework or extralaryngeal scarring, and they usually resolve to subclinical levels within weeks or months after the operation (9,13,14). Dividing the sternothyroid muscle has been shown not to affect voice outcome in a significant way (15). Even though the RLN is the most important provider of laryngeal motor innervation, injury to the external branch of the SLN is also believed to cause significant voice changes, such as reduction in the fundamental frequency range, reduction in the highest obtainable fundamental frequency and vibratory phase asymmetry in the vocal folds (16-19). The external branch of the SLN has therefore gained increased attention among thyroid surgeons, and it is recommended that it should be preserved as well as the RLN during thyroid surgery (20).
In most cases of postoperative vocal fold paresis, the RLN is macroscopically intact and the injury is located within the peripheral nerve. To be able to prognosticate recovery, the surgeon would need to define the presence, location, and type of the nerve injury. However, very little data has been presented aimed at clarifying the differences between the transient and permanent injury of the RLN. This review aims to provide, from an anatomical and neurobiological perspective, an update on the current understanding of surgically-induced injury to the laryngeal nerves.
The laryngeal nerves
The larynx is innervated by two branches of the vagus nerve, the RLN, and the SLN. Both nerves carry motor, sensory, and autonomic (parasympathetic) fibers to the larynx (21). The lower motor neurons of the special efferent system controlling the intrinsic laryngeal muscles are located in the nucleus ambiguus in the lower brainstem, in a fairly well-studied somatotopical arrangement (22-30). The sensory neurons are located in the nodose ganglion and the parasympathetic cell bodies are located in the dorsal motor nucleus of the vagus in the brainstem. The mechanically complex laryngeal functions (airway protection reflexes, phonation, swallowing) require a rich and detailed neural control, projected through the RLN and SLN.
The RLN can be regarded as the most important motor nerve supply to the larynx, as it innervates 4 out of 5 intrinsic laryngeal muscles. It also has projections to the esophagus and trachea (23). The RLN divides into an anterior and posterior branch. The branching point is located either inside the larynx, or, as in roughly one third of cases, before the nerve entering point (31). The posterior branch projects superiorly to form the anastomosis of Galen with the internal branch of the SLN—this branch is probably sensory in nature (32). The anterior branch carries motor fibers (33,34) to the posterior thyroarytenoid muscle, lateral cricoarytenoid muscle and, finally, to the thyroarytenoid muscle (35-37). Within the larynx, the RLN and SLN break up into a plexus-like branching system (38), with several connections between the RLN and SLN (37). The exact functions of these small nerve branches are not fully known, but it has been demonstrated in animal experimental models that the intrinsic laryngeal muscles receive dual innervation from both the RLN and SLN (39,40).
The SLN originates from the inferior vagal ganglion at the C2 level in the neck (41). It divides into a larger, internal branch which enters the larynx through the thyrohyoid membrane (carrying sensory fibers down to the level of the glottis) and a smaller, external, branch which passes deep to the superior thyroid artery to innervate the cricothyroid muscle responsible for vocal fold lengthening and tension, important for high voice pitch (42). The external branch of the SLN continues through the cricothyroid muscle to reach the anterior glottis and the thyroarytenoid muscle. This branch, called “the human communicating nerve” (43), or “the cricothyroid connection branch” (44) thus represents an additional motor supply to the intrinsic laryngeal muscles other than the RLN, which may be important following RLN injury and reinnervation. This anatomy enables intraoperative monitoring of the external branch of the SLN through routine surface electrodes in the intubation tube (45), although the exact laryngological function of this nerve branch is not known (17).
Neurapraxia versus axonotmesis
From a clinical perspective, it is important to make the distinction between nerve conduction block, “neurapraxia”, and the more severe “axonotmesis”, which means presence of axonal injury (Figure 1). These classifications were first made by Seddon in 1942 (46) and later modified by Sunderland in 1951 (47). Surgically-induced nerve injuries seldom include complete transection of the nerve, but rather intraneural damage inside a macroscopically intact nerve due to pressure, crush or heating from adjacent use of cautery. Neurapraxia is the mildest form of injury, affecting the surrounding Schwann cells, but respecting the integrity of the axon (Figure 1A). The result is a conduction block lasting typically about 6-8 weeks followed by a complete return of function, when the Schwann cells have been repaired (48). This seems to be the case also for RLN injury (49). Following axonotmesis, there is a varying degree of axonal injury (Figure 1B), which could lead to neuronal death or dysfunctional reinnervation of the target cells. Axonotmesis, therefore, is associated with a poorer and more unpredictable outcome for functional restitution.
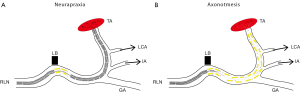
Neurobiology behind impaired function
The motor neuron terminates at the neuromuscular junction, the motor end plate. The neuron is the secondary neuron and is part of the peripheral nervous system (PNS), as opposed to the primary motor neuron [central nervous system (CNS)], which runs from the cortex to terminate on the secondary neurons. The myelin around the axons in the CNS comes from oligodendrocytes. The CNS myelin contains several factors which are inhibitory to axonal growth and regeneration, which is one of the major problems after CNS injury, such as stroke or spinal cord injury (50). In the PNS, on the other hand, the myelin around the axons is derived from the Schwann cell. This milieu is attractive for axonal growth, which is why the peripheral nerve injury is usually associated with regeneration after axonal disruption (51-55). Following a peripheral nerve injury, which involves peripheral axon disruption, the distal part (which is disconnected from the neuron) will be neurophysiologically active until it degenerates (56) [Wallerian degeneration (57)] which, under normal conditions, will take approximately one week. Thus, a complete injury to the RLN which separates the nerve into two different parts, will give a negative signal using intraoperative nerve monitoring (IONM) and the distal part a positive signal in the thyroarytenoid muscle for several days. Re-exploring the distal end of the RLN at our institution for nerve re-construction confirms the positive signal for up to five days after complete injury after thyroid surgery (unpublished observation). After axonal injury within the macroscopically intact RLN, the distal axon also degenerates and, to achieve any functional recovery, the axon has to regenerate. In the literature, there has been considerable speculation concerning the reasons for the poor (or absent) functional recovery seen after injury to the RLN despite the fact that the nerve looks macroscopically intact during surgery. One factor associated with the insufficient recovery is a potential misguidance of RLN axons during regeneration, leading to non-functional reinnervation of laryngeal muscles. There are, however, studies that show that the degree of accurate innervation is very high after crush injury to the peripheral nerve (90%) (58) since the axon is guided by intact mechanical factors of the intact endoneural tubes (59). This may reduce the impact of the misguidance as a negative factor in the injured intact RLN.
The axotomy induces a retrograde injury signal to the neuron in the brainstem which is attacked by microglia and also surrounded by a profound astroglial reaction (60-62). The neuron downregulates its production of transmitter substances and turns the gene transcription to regeneration and re-innervation. The neuron is exposed to stress and is dependent on a continuous inflow of growth factors from the periphery (54). Motor neurons are more likely to die in response to peripheral axotomy the closer the axotomy is to the neuronal soma in the brainstem or spinal cord. The more of the peripheral nerve which is in contact with the neuron soma, the more trophic support of growth factors is delivered to the neuron. The addition of growth factors radically improves the prognosis for the axotomized neuron (63-66). In addition, there are many experiments to support that distal peripheral nerve injury is associated with no or limited nerve cell death, including injury to the RLN (67).
The distal axotomy in the intact RLN also causes a synaptic displacement from the secondary motor neuron in the nucleus ambiguus, which then loses contact with higher cortical centers (68). These synapses from cortical neurons re-appear on the secondary motor neurons as the neurons manage to regenerate and re-establish contact with the target organ (muscle). The proceeding adaptation to the new neural circuits is referred to as plasticity of the nervous system. Thus, the macroscopically intact but injured RLN will recover spontaneously if there is only a conduction block caused by an impairment of electrical propagation due to Schwann cell affection. If there is a component of axonal injury within the nerve, the axon will not only have to re-innervate the laryngeal muscles, but the neuron in the brainstem will need a re-connection with cortical neurons by re-establishment of their synapses onto the secondary neuron in the brainstem.
Intraoperative nerve monitoring (IONM)
IONM of the RLN is performed by stimulating the peripheral nerve directly with an electrical current, with subsequent recording of muscle depolarization of the thyroarytenoid muscle. When a peripheral nerve is directly stimulated at supramaximal intensity, the result is depolarization of all axons and activation of all motor units projecting through the nerve, which leads to acetylcholine-mediated depolarization of muscle fibers. The intramuscular shift in electrical potential (voltage) can be recorded as a compound muscle action potential (CMAP), representing the sum of all motor unit activity. During IONM, the presence and the amplitude of the CMAP is utilized as an indirect measurement of motor nerve function during the surgery. Originally, RLN monitoring was made through needle electrodes inserted into the intrinsic laryngeal muscles. In modern clinical routine, thyroarytenoid depolarization is recorded through surface electrodes on the ventilation tube (69).
Manipulation of the surgical field may affect the RLN by traction, heating, entrapment or squeezing (crush injury), which leads to absence or reduced amplitude of the recorded CMAP following vagal stimulation. The neurobiological explanation for reduced CMAP amplitude is simply that a lower number of axons are transmitting the electrical signal, which means less depolarization of the monitored muscle. Provided that the vagal stimulation is performed in the same way [consistent with continuous monitoring (70)], the site of injury could be anywhere along the nerve, distal to the site of stimulation. When the signal is lost during surgery, it is not possible to use IONM to diagnose the type of nerve injury (axonotmesis or neurapraxia), it only tells us that there is a discontinuity of the electrical propagation within the nerve.
In order to categorize and group injuries to the RLN using IONM, one reported way is to define the injury to the RLN and loss of signal (LOS) from the vagal nerve as segmental (type 1) or global (type 2) (69,71). Looking at the basic neurobiology of the nerve, a LOS could originate from an injury anywhere from the stimulus (vagal nerve) to the neuromuscular endplate, including the muscle. The distal part of the nerve is excitable for several days after injury even after nerve transection injury (48), which makes it possible for the surgeon to pin-point the exact location of the nerve injury, by using the stimulation probe along the course of the peripheral nerve. A neurobiological explanation for the “global” (type 2) RLN injury could be that the location of the nerve injury is located distal to the nerve entry point under the inferior constrictor muscle, not affecting the whole neuron (i.e., a milder form of injury). From this perspective, type 1 and 2 injuries describe if the RLN conduction block is proximal or distal to the RLN/cricothyroid border (Figure 2).
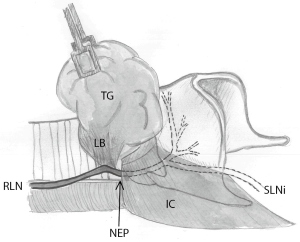
Laryngeal EMG
Postoperative electrodiagnostic methods can be used to determine the presence and type of nerve injury, as well as to characterize the ongoing or completed reinnervation processes. Laryngeal electromyography (LEMG) was first introduced more than sixty years ago, and has evolved (72) into a valuable tool for laryngologists in diagnosing neurolaryngological disorders. It has been pointed out that LEMG is primarily a qualitative method (73) (presence of denervation potentials, degree of motor unit recruitment), which makes it a subjective test depending on the examiner and the technical settings. But LEMG has nevertheless been shown to have high positive predictive value in predicting the long-term outcome of patients with a poor prognosis (74-77) and it is used widely to predict recovery regardless of the etiology behind the vocal fold paresis. Patients with pathological electromyographic findings at least two months after the paresis are most likely to need laryngeal framework surgery (76). In the case of postoperative vocal fold paresis after thyroid/parathyroid surgery, the prognostic information obtained from LEMG can be helpful to identify those cases where future interventions are necessary, which could mean surgical or pharmacological reinnervation therapies, or vocal fold medialization procedures. For patients with only a conduction block (neurapraxia) of the RLN, vocal fold movement is most likely to return. When using LEMG to obtain this information after thyroid surgery, it is important to take into consideration the timing of the examination. Denervation activity (indicating axonotmesis and poor prognosis) typically appears at three weeks after the RLN injury (48), and lasts until reinnervation is complete. Reinnervation of the intrinsic laryngeal muscles can be expected to take place rather promptly, given the high regenerative capacity of the RLN (78), together with collateral reinnervation by adjacent, intact nerve fibers (40). The optimal time window for postoperative LEMG seems to be 2-4 weeks after the nerve injury (49). Interpretation and analysis of electrophysiological data requires the expertise of a trained neurologist or clinical neurophysiologist, while insertion of the needle electrodes into the appropriate intrinsic laryngeal muscles is best performed by an ENT specialist. LEMG thus requires the cooperation and coordination of different clinical resources. A consensus paper for LEMG guidelines in the areas of indications, technical considerations, implementations and data interpretation was published by Volk et al. (79) in 2012.
Regeneration promotion
A mixed injury of demyelination (neurapraxia) and axonotmesis within the macroscopically intact RLN has a worse prognosis than demyelination alone, because of the need for regeneration and reinnervation of the target. Reinnervation of the intrinsic laryngeal muscles following axonotmesis is considered problematic (80), due to misguided, unorderly regeneration and perhaps also collateral reinnervation originating from adjacent, intact nerve fibers (40). Pathological reinnervation leads to a change in the somatotopic map, not in line with normal vocal fold function. Theoretically, it would be beneficial for the functional restitution to pace up and enhance regeneration/reinnervation by the RLN.
In vitro, it has been shown that the pace of the regenerating axon is regulated at the tip (growth cone), the motion of which is highly dependent on a delicate regulation of calcium ions (81,82). It was demonstrated that altering the intracellular concentration of calcium ions had a strict correlation to the ability of the growth cone to sprout (82). The regulation of intracellular calcium is also closely linked to the actions of the voltage-gated calcium channels present in the cellular membrane (82). In vivo, it has been confirmed that the transient quick calcium currents across the membrane of the growth cone occur with a certain frequency. If the calcium transient calcium currents are to some extent inhibited the pace of axonal elongation increases, and vice versa (83-85). In fact, blocking of the rapid calcium flow current across the membrane would increase the total time for axonal elongation, a principle further evaluated in experimental models. Nimodipine, a voltage-gated calcium flow antagonist to the L-type channels has been evaluated in rodent models, and is a pharmacologically good choice because it penetrates the blood brain barrier better than most other calcium flow antagonists (86). After systemic administration of nimodipine, an improved regeneration and functional recovery has experimentally been achieved after injury to the sciatic (87), facial (88-90), hypoglossal (91) and RLNs (92). In the patient, nimodipine has been evaluated after recurrent laryngeal (49,93-95) and facial nerve injury (96-100), with promising functional outcomes. Taken together, there is substantial evidence that the administration of nimodipine after axonal injury to a peripheral nerve probably improves the functional outcome.
Even though there is emerging data that treatment with nimodipine may also be translated to the patient in some situations, the level of evidence for a using nimodipine for intraoperative RLN injury is still modest. Only a fraction of the patients with postoperative RLN paresis would benefit from a regeneration-promoting treatment (i.e., cases with axonotmesis). It is important, therefore, to search for further knowledge concerning diagnosis and prognosis after RLN injury after thyroid surgery.
Conclusions
Laryngeal dysfunction and voice problems are common after thyroid surgery, but only a fraction of these cases turn out to be chronic. Chronic laryngeal dysfunction is most commonly caused by axonal injury to the RLN or SLN. The clinical progress of symptoms and the eventual functional recovery of the target organ follow the general principles of peripheral nerve injury, even though the larynx can be regarded a special case in being functionally and neuroanatomically complex, with high demands for accurate neural supply. Today, it is possible for the clinician to utilize the information obtained from electrodiagnostic methods (IONM and postoperative LEMG), to characterize the nerve injury and predict the temporal course and functional result of the healing process. It is important to do so in order to be prepared for additional interventions, such as voice therapy, medialization surgery or regeneration/reinnervation therapies.
Acknowledgements
Disclosure: The authors declare no conflict of interest.
References
- Collazo-Clavell ML, Gharib H, Maragos NE. Relationship between vocal cord paralysis and benign thyroid disease. Head Neck 1995;17:24-30. [PubMed]
- Farrag TY, Samlan RA, Lin FR, et al. The utility of evaluating true vocal fold motion before thyroid surgery. Laryngoscope 2006;116:235-8. [PubMed]
- Randolph GW, Kamani D. The importance of preoperative laryngoscopy in patients undergoing thyroidectomy: voice, vocal cord function, and the preoperative detection of invasive thyroid malignancy. Surgery 2006;139:357-62. [PubMed]
- Shaha AR. Routine laryngoscopy in thyroid surgery: A valuable adjunct. Surgery 2007;142:865-6. [PubMed]
- Jeannon JP, Orabi AA, Bruch GA, et al. Diagnosis of recurrent laryngeal nerve palsy after thyroidectomy: a systematic review. Int J Clin Pract 2009;63:624-9. [PubMed]
- Francis DO, Pearce EC, Ni S, et al. Epidemiology of vocal fold paralyses after total thyroidectomy for well-differentiated thyroid cancer in a Medicare population. Otolaryngol Head Neck Surg 2014;150:548-57. [PubMed]
- Kuhn MA, Bloom G, Myssiorek D. Patient perspectives on dysphonia after thyroidectomy for thyroid cancer. J Voice 2013;27:111-4. [PubMed]
- Vicente DA, Solomon NP, Avital I, et al. Voice outcomes after total thyroidectomy, partial thyroidectomy, or non-neck surgery using a prospective multifactorial assessment. J Am Coll Surg 2014;219:152-63. [PubMed]
- Page C, Zaatar R, Biet A, et al. Subjective voice assessment after thyroid surgery: a prospective study of 395 patients. Indian J Med Sci 2007;61:448-54. [PubMed]
- Hong KH, Kim YK. Phonatory characteristics of patients undergoing thyroidectomy without laryngeal nerve injury. Otolaryngol Head Neck Surg 1997;117:399-404. [PubMed]
- Akyildiz S, Ogut F, Akyildiz M, et al. A multivariate analysis of objective voice changes after thyroidectomy without laryngeal nerve injury. Arch Otolaryngol Head Neck Surg 2008;134:596-602. [PubMed]
- Debruyne F, Ostyn F, Delaere P, et al. Acoustic analysis of the speaking voice after thyroidectomy. J Voice 1997;11:479-82. [PubMed]
- Maeda T, Saito M, Otsuki N, et al. Voice quality after surgical treatment for thyroid cancer. Thyroid 2013;23:847-53. [PubMed]
- Minni A, Ruoppolo G, Barbaro M, et al. Long-term (12 to 18 months) functional voice assessment to detect voice alterations after thyroidectomy. Eur Rev Med Pharmacol Sci 2014;18:1704-8. [PubMed]
- Henry LR, Solomon NP, Howard R, et al. The functional impact on voice of sternothyroid muscle division during thyroidectomy. Ann Surg Oncol 2008;15:2027-33. [PubMed]
- Mendelsohn AH, Sung MW, Berke GS, et al. Strobokymographic and videostroboscopic analysis of vocal fold motion in unilateral superior laryngeal nerve paralysis. Ann Otol Rhinol Laryngol 2007;116:85-91. [PubMed]
- Orestes MI, Chhetri DK. Superior laryngeal nerve injury: effects, clinical findings, prognosis, and management options. Curr Opin Otolaryngol Head Neck Surg 2014;22:439-43. [PubMed]
- Roy N, Barton ME, Smith ME, et al. An in vivo model of external superior laryngeal nerve paralysis: laryngoscopic findings. Laryngoscope 2009;119:1017-32. [PubMed]
- Roy N, Smith ME, Dromey C, et al. Exploring the phonatory effects of external superior laryngeal nerve paralysis: an in vivo model. Laryngoscope 2009;119:816-26. [PubMed]
- Chandrasekhar SS, Randolph GW, Seidman MD, et al. Clinical practice guideline: improving voice outcomes after thyroid surgery. Otolaryngol Head Neck Surg 2013;148:S1-37. [PubMed]
- Gacek RR, Lyon MJ. Fiber components of the recurrent laryngeal nerve in the cat. Ann Otol Rhinol Laryngol 1976;85:460-71. [PubMed]
- Berkowitz RG, Sun QJ, Chalmers J, et al. Identification of posterior cricoarytenoid motoneurons in the rat. Ann Otol Rhinol Laryngol 1999;108:1033-41. [PubMed]
- Bieger D, Hopkins DA. Viscerotopic representation of the upper alimentary tract in the medulla oblongata in the rat: the nucleus ambiguus. J Comp Neurol 1987;262:546-62. [PubMed]
- Gacek RR. Localization of laryngeal motor neurons in the kitten. Laryngoscope 1975;85:1841-61. [PubMed]
- Gacek RR, Malmgren LT. Laryngeal motor innervation-central. In: Blitzer A, Brin MF, Sasaki CT, et al. eds. Neurologic disorders of the larynx. New York: Thieme, 1992:29-35.
- Hinrichsen CF, Ryan AT. Localization of laryngeal motoneurons in the rat: morphologic evidence for dual innervation? Exp Neurol 1981;74:341-55. [PubMed]
- Kobler JB, Datta S, Goyal RK, et al. Innervation of the larynx, pharynx, and upper esophageal sphincter of the rat. J Comp Neurol 1994;349:129-47. [PubMed]
- Lobera B, Pásaro R, González-Barón S, et al. A morphological study of ambiguus nucleus motoneurons innervating the laryngeal muscles in the rat and cat. Neurosci Lett 1981;23:125-30. [PubMed]
- Patrickson JW, Smith TE, Zhou SS. Motor neurons of the laryngeal nerves. Anat Rec 1991;230:551-6. [PubMed]
- Portillo F, Pásaro R. Location of motoneurons supplying the intrinsic laryngeal muscles of rats. Horseradish peroxidase and fluorescence double-labeling study. Brain Behav Evol 1988;32:220-5. [PubMed]
- Fontenot TE, Randolph GW, Friedlander PL, et al. Gender, race, and electrophysiologic characteristics of the branched recurrent laryngeal nerve. Laryngoscope 2014;124:2433-7. [PubMed]
- Sandillon H. eds. Le role de l'anse de Galien Unités d'Enseignement et de Recherche des Siences Médicales. Bordeaux: Université de Bordeaux II, 1984.
- Kandil E, Abdelghani S, Friedlander P, et al. Motor and sensory branching of the recurrent laryngeal nerve in thyroid surgery. Surgery 2011;150:1222-7. [PubMed]
- Serpell JW, Yeung MJ, Grodski S. The motor fibers of the recurrent laryngeal nerve are located in the anterior extralaryngeal branch. Ann Surg 2009;249:648-52. [PubMed]
- Maranillo E, León X, Ibañez M, et al. Variability of the nerve supply patterns of the human posterior cricoarytenoid muscle. Laryngoscope 2003;113:602-6. [PubMed]
- Maranillo E, Leon X, Orus C, et al. Variability in nerve patterns of the adductor muscle group supplied by the recurrent laryngeal nerve. Laryngoscope 2005;115:358-62. [PubMed]
- Sañudo JR, Maranillo E, León X, et al. An anatomical study of anastomoses between the laryngeal nerves. Laryngoscope 1999;109:983-7. [PubMed]
- Dilworth TF. The Nerves of the Human Larynx. J Anat 1921;56:48-52. [PubMed]
- Björck G, Margolin G, Måbäck GM, et al. New animal model for assessment of functional laryngeal motor innervation. Ann Otol Rhinol Laryngol 2012;121:695-9. [PubMed]
- Hydman J, Mattsson P. Collateral reinnervation by the superior laryngeal nerve after recurrent laryngeal nerve injury. Muscle Nerve 2008;38:1280-9. [PubMed]
- Monfared A, Kim D, Jaikumar S, et al. Microsurgical anatomy of the superior and recurrent laryngeal nerves. Neurosurgery 2001;49:925-32; discussion 932-3. [PubMed]
- Shaw GY, Searl JP, Hoover LA. Diagnosis and treatment of unilateral cricothyroid muscle paralysis with a modified Isshiki type 4 thyroplasty. Otolaryngol Head Neck Surg 1995;113:679-88. [PubMed]
- Wu BL, Sanders I, Mu L, et al. The human communicating nerve. An extension of the external superior laryngeal nerve that innervates the vocal cord. Arch Otolaryngol Head Neck Surg 1994;120:1321-8. [PubMed]
- Maranillo E, León X, Quer M, et al. Is the external laryngeal nerve an exclusively motor nerve? The cricothyroid connection branch. Laryngoscope 2003;113:525-9. [PubMed]
- Barczyński M, Randolph GW, Cernea CR, et al. External branch of the superior laryngeal nerve monitoring during thyroid and parathyroid surgery: International Neural Monitoring Study Group standards guideline statement. Laryngoscope 2013;123 Suppl 4:S1-14. [PubMed]
- Seddon HJ. A Classification of Nerve Injuries. Br Med J 1942;2:237-9. [PubMed]
- Sunderland S. A classification of peripheral nerve injuries producing loss of function. Brain 1951;74:491-516. [PubMed]
- Kimura J. eds. Electrodiagnosis in Diseases of Nerve and Muscle: Principles and Practices. 3rd ed. Oxford: Oxford University Press, 2001.
- Hydman J, Björck G, Persson JK, et al. Diagnosis and prognosis of iatrogenic injury of the recurrent laryngeal nerve. Ann Otol Rhinol Laryngol 2009;118:506-11. [PubMed]
- Schwab ME. Nogo and axon regeneration. Curr Opin Neurobiol 2004;14:118-24. [PubMed]
- DeFrancesco-Lisowitz A, Lindborg JA, Niemi JP, et al. The neuroimmunology of degeneration and regeneration in the peripheral nervous system. Neuroscience 2014. [Epub ahead of print]. [PubMed]
- Fawcett JW, Keynes RJ. Peripheral nerve regeneration. Annu Rev Neurosci 1990;13:43-60. [PubMed]
- Ide C. Peripheral nerve regeneration. Neurosci Res 1996;25:101-21. [PubMed]
- Richner M, Ulrichsen M, Elmegaard SL, et al. Peripheral nerve injury modulates neurotrophin signaling in the peripheral and central nervous system. Mol Neurobiol 2014;50:945-70. [PubMed]
- Scheib J, Höke A. Advances in peripheral nerve regeneration. Nat Rev Neurol 2013;9:668-76. [PubMed]
- Cajal YR. eds. Degeneration and regeneration of the nervous system. Oxford: Oxford University Press, 1928.
- Waller A. Experiments on the section of the glossopharyngeal and hypoglossal nerves of the frog, and observations of the alterations produced thereby in the structure of their primitive fibers. Philos Trans R Soc London (Biol) 1850;140:423-29.
- Fournier AE, Strittmatter SM. Regenerating nerves follow the road more traveled. Nat Neurosci 2002;5:821-2. [PubMed]
- Nguyen QT, Sanes JR, Lichtman JW. Pre-existing pathways promote precise projection patterns. Nat Neurosci 2002;5:861-7. [PubMed]
- Aldskogius H, Svensson M. Neuronal and glial cell responses to axon injury. Advances in Structural Biology 1993;2:191-223.
- Svensson M, Aldskogius H. The effect of axon injury on microtubule-associated proteins MAP2, 3 and 5 in the hypoglossal nucleus of the adult rat. J Neurocytol 1992;21:222-31. [PubMed]
- Svensson M, Eriksson P, Persson JK, et al. The response of central glia to peripheral nerve injury. Brain Res Bull 1993;30:499-506. [PubMed]
- Oppenheim RW, Houenou LJ, Johnson JE, et al. Developing motor neurons rescued from programmed and axotomy-induced cell death by GDNF. Nature 1995;373:344-6. [PubMed]
- Oppenheim RW, Yin QW, Prevette D, et al. Brain-derived neurotrophic factor rescues developing avian motoneurons from cell death. Nature 1992;360:755-7. [PubMed]
- Sendtner M, Holtmann B, Kolbeck R, et al. Brain-derived neurotrophic factor prevents the death of motoneurons in newborn rats after nerve section. Nature 1992;360:757-9. [PubMed]
- Sendtner M, Kreutzberg GW, Thoenen H. Ciliary neurotrophic factor prevents the degeneration of motor neurons after axotomy. Nature 1990;345:440-1. [PubMed]
- Hydman J, Svensson M, Kuylenstierna R, et al. Neuronal survival and glial reactions after recurrent laryngeal nerve resection in the rat. Laryngoscope 2005;115:619-24. [PubMed]
- Blinzinger K, Kreutzberg G. Displacement of synaptic terminals from regenerating motoneurons by microglial cells. Z Zellforsch Mikrosk Anat 1968;85:145-57. [PubMed]
- Randolph GW, Dralle H. International Intraoperative Monitoring Study Group, Electrophysiologic recurrent laryngeal nerve monitoring during thyroid and parathyroid surgery: international standards guideline statement. Laryngoscope 2011;121 Suppl 1:S1-16. [PubMed]
- Dionigi G, Donatini G, Boni L, et al. Continuous monitoring of the recurrent laryngeal nerve in thyroid surgery: a critical appraisal. Int J Surg 2013;11 Suppl 1:S44-6. [PubMed]
- Phelan E, Schneider R, Lorenz K, et al. Continuous vagal IONM prevents recurrent laryngeal nerve paralysis by revealing initial EMG changes of impending neuropraxic injury: a prospective, multicenter study. Laryngoscope 2014;124:1498-505. [PubMed]
- Faaborg-andersen K, Buchthal F. Action potentials from internal laryngeal muscles during phonation. Nature 1956;177:340-1. [PubMed]
- Blitzer A, Crumley RL, Dailey SH, et al. Recommendations of the Neurolaryngology Study Group on laryngeal electromyography. Otolaryngol Head Neck Surg 2009;140:782-93. [PubMed]
- Rickert SM, Childs LF, Carey BT, et al. Laryngeal electromyography for prognosis of vocal fold palsy: a meta-analysis. Laryngoscope 2012;122:158-61. [PubMed]
- Smith LJ, Rosen CA, Niyonkuru C, et al. Quantitative electromyography improves prediction in vocal fold paralysis. Laryngoscope 2012;122:854-9. [PubMed]
- Wang CC, Chang MH, De Virgilio A, et al. Laryngeal electromyography and prognosis of unilateral vocal fold paralysis-A long-term prospective study. Laryngoscope 2014. [Epub ahead of print]. [PubMed]
- Wang CC, Chang MH, Wang CP, et al. Prognostic indicators of unilateral vocal fold paralysis. Arch Otolaryngol Head Neck Surg 2008;134:380-8. [PubMed]
- Crumley RL. Repair of the recurrent laryngeal nerve. Otolaryngol Clin North Am 1990;23:553-63. [PubMed]
- Volk GF, Hagen R, Pototschnig C, et al. Laryngeal electromyography: a proposal for guidelines of the European Laryngological Society. Eur Arch Otorhinolaryngol 2012;269:2227-45. [PubMed]
- Crumley RL. Laryngeal synkinesis revisited. Ann Otol Rhinol Laryngol 2000;109:365-71. [PubMed]
- Kater SB, Mattson MP, Cohan C, et al. Calcium regulation of the neuronal growth cone. Trends Neurosci 1988;11:315-21. [PubMed]
- Kater SB, Mills LR. Regulation of growth cone behavior by calcium. J Neurosci 1991;11:891-9. [PubMed]
- Gomez TM, Spitzer NC. In vivo regulation of axon extension and pathfinding by growth-cone calcium transients. Nature 1999;397:350-5. [PubMed]
- Gomez TM, Spitzer NC. Regulation of growth cone behavior by calcium: new dynamics to earlier perspectives. J Neurobiol 2000;44:174-83. [PubMed]
- Gomez TM, Zheng JQ. The molecular basis for calcium-dependent axon pathfinding. Nat Rev Neurosci 2006;7:115-25. [PubMed]
- Van den Kerckhoff W, Drewes LR. Transfer of the calcium antagonists nifedipine and nimodipine across the blood brain barrier and their regional distribution in vivo. J Cerebr Blood Flow Metab 1985; 5 Suppl 1:459-60.
- van der Zee CE, Schuurman T, Traber J, et al. Oral administration of nimodipine accelerates functional recovery following peripheral nerve damage in the rat. Neurosci Lett 1987;83:143-8. [PubMed]
- Angelov DN, Neiss WF, Streppel M, et al. Nimodipine accelerates axonal sprouting after surgical repair of rat facial nerve. J Neurosci 1996;16:1041-8. [PubMed]
- Mattsson P, Aldskogius H, Svensson M. Nimodipine-induced improved survival rate of facial motor neurons following intracranial transection of the facial nerve in the adult rat. J Neurosurg 1999;90:760-5. [PubMed]
- Mattsson P, Janson AM, Aldskogius H, et al. Nimodipine promotes regeneration and functional recovery after intracranial facial nerve crush. J Comp Neurol 2001;437:106-17. [PubMed]
- Angelov DN, Neiss WF, Gunkel A, et al. Nimodipine-accelerated hypoglossal sprouting prevents the postoperative hyperinnervation of target muscles after hypo glossal-facial anastomosis in the rat. Restor Neurol Neurosci 1997;11:109-21. [PubMed]
- Hydman J, Remahl S, Björck G, et al. Nimodipine improves reinnervation and neuromuscular function after injury to the recurrent laryngeal nerve in the rat. Ann Otol Rhinol Laryngol 2007;116:623-30. [PubMed]
- Mattsson P, Björck G, Remahl S, et al. Nimodipine and microsurgery induced recovery of the vocal cord after recurrent laryngeal nerve resection. Laryngoscope 2005;115:1863-5. [PubMed]
- Rosen CA, Smith L, Young V, et al. Prospective investigation of nimodipine for acute vocal fold paralysis. Muscle Nerve 2014;50:114-8. [PubMed]
- Sridharan SS, Rosen CA, Smith LJ, et al. Timing of nimodipine therapy for the treatment of vocal fold paralysis. Laryngoscope 2015;125:186-90. [PubMed]
- Scheller C, Richter HP, Engelhardt M, et al. The influence of prophylactic vasoactive treatment on cochlear and facial nerve functions after vestibular schwannoma surgery: a prospective and open-label randomized pilot study. Neurosurgery 2007;61:92-7; discussion 97-8. [PubMed]
- Scheller C, Wienke A, Wurm F, et al. Neuroprotective efficacy of prophylactic enteral and parenteral nimodipine treatment in vestibular schwannoma surgery: a comparative study. J Neurol Surg A Cent Eur Neurosurg 2014;75:251-8.
- Scheller K, Scheller C. Nimodipine promotes regeneration of peripheral facial nerve function after traumatic injury following maxillofacial surgery: an off label pilot-study. J Craniomaxillofac Surg 2012;40:427-34. [PubMed]
- Scheller K, Scheller C. Nimodipine for peripheral nerve recovery after maxillofacial and vestibular schwannoma surgery. Muscle Nerve 2014;50:1026-7. [PubMed]
- Strauss C, Romstöck J, Fahlbusch R, et al. Preservation of facial nerve function after postoperative vasoactive treatment in vestibular schwannoma surgery. Neurosurgery 2006;59:577-84; discussion 577-84. [PubMed]