Safety of Ligasure exact dissector in thyroidectomy with continuous neuromonitoring: a porcine model
Introduction
In thyroid surgery, meticulous hemostasis is essential for preventing postoperative bleeding, which may cause life-threatening complications (1). Hemostatic methods developed so far include clamp-and-tie technique, suture ligation, and monopolar or bipolar electrocautery (2,3). In recent years, newly developed energy-based devices (EBDs) have been widely discussed in the literature because of their superior performance in terms of pain, operative time, hospital stay, blood loss, postoperative drainage, and incidence of hypocalcemia (4-10). Thermal injury is the second most common mechanism of recurrent laryngeal nerve (RLN) injury during thyroid surgery after traction injury (11,12). In comparison with electrocautery, the advantage of EBDs is that they do not pass electrical energy through the body. However, the high temperatures generated by EBDs put nerves at risk for thermal injury. Although EBDs have proven effective in hemostasis, a clear understanding of their safety is mandatory.
The LigasureTM exact dissector (LED) (LF2019; Medtronic) provides thermal sealing with a tissue divider and is suitable for dissection and hemostasis in thyroidectomy, similar to the LigasureTM Small Jaw (LSJ, LF1212 Europe, LF1212A USA; Medtronic). By denaturizing collagen and elastin within vessels, it can seal vessels up to 7 mm in diameter with their surrounding connective tissue (11). Measured at the tip, the LED has a jaw width of 2 mm [smaller than the jaw width of the Ligasure Small Jaw (LSJ)], and a seal length of 20.6 mm (longer than the 16.5 mm seal length of LSJ). The thinner jaw width and the longer seal length of the LED enable more precise dissection compared to LSJ.
The aim of this study was to use a porcine model to investigate safety parameters for using LED for dissection in thyroidectomy. For this purpose, we monitored the time of electromyography (EMG) change under continuous intraoperative neuromonitoring (CIONM) with the LED used at various distances from the RLN (activation study). The effects of various cooling durations and cooling maneuvers were also compared during application of LED near the RLN after activation (cooling study).
Methods
Subject preparation and anesthesia
The porcine model used in this prospective study was developed by our research team (13) and is well established in CIONM research. The animal-use protocol was consistent with national/international regulations and guidelines for animal experiments, including replacement, reduction, and refinement principles, and was approved by the Institutional Animal Care and Use Committee of Kaohsiung Medical University, Taiwan (IACUC protocol No. 108084).
In the LED experiments, endotracheal surface electrodes were used to record EMG signals evoked by electrical stimulation of vocalis muscles near the RLN.
Anesthesia was initiated by administrating 2 mg/kg of tiletamine/zolazepam intramuscularly 30 minutes before the experiment. Muscle relaxants were not used during anesthesia to avoid neuromuscular blockade that could have interfered with EMG signals during neural monitoring. After the piglets were intubated, tidal volume was set to 8–12 mL/kg, and respiratory rate was set to 15 to 20 breaths per minute. General anesthesia was maintained with sevoflurane 1% to 2%.
Equipment setting and operation
All neural monitoring procedures were performed in accordance with the guidelines of the International Neural Monitoring Study Groups (14). A nerve integrity monitor size #6 EMG endotracheal tube (NIM Standard Tube, Medtronic, Jacksonville, FL, USA) was used in the manner routinely used in humans (15). The CIONM was performed using the Nerve Integrity Monitor system (NIM3.0, Medtronic). Automated periodic stimulation (APS) of the vagus nerve (VN) was set for accurate and efficient real-time recording of EMG signals during RLN injury (Figure 1).
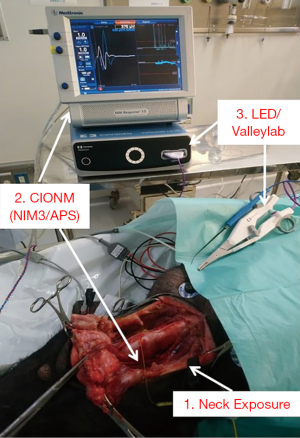
A long transverse cervical incision was made, and subcutaneous tissue and muscles were divided and retracted away from midline. The lateral border of the sternocleidomastoid (SCM) muscle was dissected and retracted medially. The thyroid glands, VNs and the RLNs were exposed adequately (Figure 1). The VN was approached laterally, dissected free of fascia, and kept dry for proper stimulation. The APS electrode was placed on the VN at the fifth tracheal ring level with optimal stability. Baseline data for VNs, including amplitude and latency of the evoked response, were calibrated automatically. An adverse EMG change was defined as a 50% decrease in amplitude or 10% increase in latency.
Figure 1 shows the LED and the ValleylabTM LS10 energy platform (Medtronic, Minneapolis, MN, USA) used as the generator in this study.
Study design
The activation study determined the distance from the RLN at which the LED can be safely used. The cooling study determined the cooling time (after prior activation) needed for safe use of the LED near the RLN. When a substantial adverse EMG change occurred, the LED was deactivated, and the continuously monitored EMG was recorded for at least 20 minutes to observe recovery of the electrophysiological response.
Activation study
Figure 2 shows the design of the activation study. The LED was applied to soft tissue in a single activation of 2–4 seconds and at a distance of 5 mm from the RLN. If no adverse EMG event (e.g., significant adverse amplitude decrease or latency increase) occurred, the distance was progressively decreased to 2, 1, and 0 mm. Tests proceeded from the proximal to the distal part of the RLN. Real-time EMG CIONM information during each LED activation was continuously recorded.
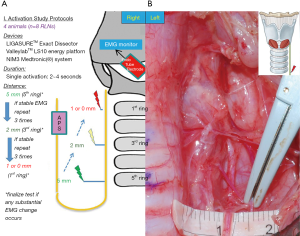
Cooling study
Figure 3 shows the design of the cooling study. First, the LED was applied to the SCM muscle in a single activation of 2 to 4 seconds then allowed to cool for 5 seconds at room temperature. The blade of the LED was then applied to the RLN. If no adverse EMG event occurred, cooling time was shortened to 2 seconds and then 0 seconds. To test the cooling effect of the “muscle touch maneuver”, the operator activated the LED on the SCM muscle and then touched another part of the SCM muscle. The blade was then allowed to cool for varying time intervals before the operator touched the RLN. In the pre-test, the duration of the muscle touch maneuver was progressively decreased from 5 seconds, to 3 seconds, and to 1 second. No EMG changes occurred. After a single activation of the LED on the SCM muscle, we immediately performed muscle touch maneuver. With the blade in full contact with the SCM at another position, the LED immediately detached from the muscle and touched the RLN. The cooling effect of double activation was also tested; after two consecutive LED activations, the operator immediately touched the RLN with the LED blade without a cooling time. The muscle touch maneuver was also tested after double activation. Real-time EMG information for each stimulation was recorded and analyzed.

Results
Anesthesia, surgery, and CIONM were successfully performed in all animals. Tables 1,2 summarize the results of the LED activation and cooling experiments.
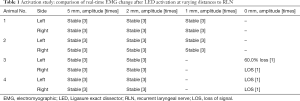
Full table
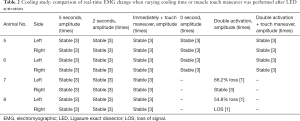
Full table
Activation study
The activation study was performed in eight RLNs of four piglets (Table 1). In tests of activation at distances of 5, 2 and 1 mm, no adverse effects, including amplitude decreases and prolonged latency, occurred. With the blade in direct contact, the left RLN of piglet 3 showed a 60.0% loss of signal (LOS) after a 20-minute observation, and three other nerves in piglets 3 and 4 (Figure 4A) showed LOS without recovery.
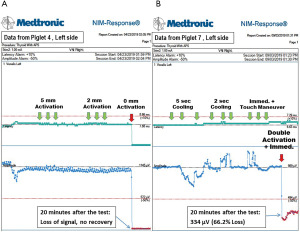
Cooling study
Table 2 shows the details of the cooling study performed in eight RLNs of four piglets. After an LED activation time of 5 seconds and a cooling time of 5 or 2 seconds, no piglets showed adverse effects when the RLN was touched with the LED. When the RLN was touched immediately after muscle touch maneuver, no piglets showed adverse effects in the RLN.
When the cooling study was performed in four nerves in piglets 5 and 6 with single activation and cooling time of 0 seconds, none of the four nerves showed adverse effects. When double activation was performed with a cooling time of 0 seconds, the left RLNs in piglets 7 and 8 showed 66.2% (Figure 4B) and 54.8% LOS, respectively; the right RLN in piglet 7 showed no adverse neural effect; the right RLN in piglet 8 showed LOS without recovery over a 20-minute observation time. When the muscle touch maneuver was performed after double activation (piglets 5 and 6), no neural side effect occurred.
Discussion
Thermal injury is a serious nerve injury mechanism that may not be easily recognized by visual inspection and can occur without direct contact with the heat source (16,17). A thermal injury resulting from a temperature exceeding 60 degrees Celsius causes permanent functional damage to the endoneurium whereas traction injury is usually limited to the perineurium and epineurium (18). After activation, most EBDs reach temperatures exceeding 60 degrees Celsius. That is, use of EBDs for dissection near nerves during surgery is a potential cause of thermal injury of varying severity. This study investigated the thermal spread of LEDs and qualified and quantified their safety parameters.
Intraoperative neuromonitoring is increasingly used to identify the RLN during thyroid surgery. Neuromonitoring is helpful for detecting nerve injury mechanisms and for predicting postoperative vocal cord function (14,19). By using repetitive vagal stimulation to provide continuous visual and acoustic feedback for nerve function, CIONM enables early detection of nerve injury after high risk procedures (20-22). For example, using CIONM to identify a nerve traction injury mechanism at an early stage, enables corrective action when preservation of function is still possible. However, since thermal injuries to nerves often occur suddenly and unexpectedly, correcting the nerve injury mechanism is usually difficult, and recovery is rare (18,23). The LED is a newly designed device that increases the precision of dissection during thyroid surgery. Compared to LSJ, the thinner tip of the LED enables insertion of the tip into small spaces (e.g., ligament of Berry region). The generator (ValleylabTM LS10 Energy Platform) used in the LED also differs from that in the LSJ (ForceTriad™ Energy Platform). The generators for the LED and LSJ have peak-to-peak voltages of 500 and 5,785 V at 1 kΩ, rated loads of 30 and 20 Ω, and maximum power of 270 and 350 Watts, respectively. Notably, LED can provide adequate vessel sealing with a shorter activation time compared to LSJ. In our experiments, LED also achieved hemostasis faster compared to LSJ (17). A single activation of LED required 2 to 4 seconds. According to the specifications listed on its official website, the jaw temperature after one activation is less than 60 degrees Celsius. After 5 activations, the jaw cools to 60 degrees Celsius within 10 seconds. A shorter activation time and a lower activation temperature may reduce thermal injury in LED use. Activation with immediate contact still caused signal loss in most nerves. The portion of the blade without heat insulation can still cause thermal injury to nerves during dissection. In our study, the temperature was much higher after a double activation compared to a single activation, and thermal injuries occurred when no cooling time was allowed. Muscle touch maneuver after double activation did not cause thermal injury in our experiments. Use of LED in actual surgery still requires the muscle touch maneuver before dissection of the nerve area after repeated hemostasis.
Some limitations of this study are noted. First, some aspects of this prospective porcine model and some data for the animal study may not be applicable to human surgery. Additionally, several factors can affect heat transfer from the LED to the RLN, including operating room conditions and the difference between human and experimental animals. However, this model has proven useful and reliable for depicting real-time changes in laryngeal EMG in surgery for RLN injury (12,16-18,24,25). Second, this study focused on real-time EMG changes during a 20-minute period since RLN injury, and which was lack of long-term RLN function outcomes. Notably, the accuracy of CIONM data for predicting future vocal cord function is well established (22,26). Finally, it should be noted that soft tissue coagulation may require use of the LED at a shorter distance to the RLN. Therefore, although this study indicates that the minimum safe distance for LED is 1 mm, the tip should be closely monitored during surgery, and activation should be stopped immediately if soft tissue coagulation causes the nerve to be pulled close to the tip during dissection near the RLN.
Conclusions
Since LED may cause iatrogenic RLN thermal injury, standard procedures for its use must be developed and applied. The RLN should be clearly visualized, and the LED must be kept at a minimum distance of 1 mm from the nerve for safe use. When using the LED for dissection near the RLN, an adequate cooling time after activation and muscle touch maneuver are helpful for preventing RLN injury.
Acknowledgments
Funding: This study was supported by grants from Kaohsiung Medical University Hospital, Kaohsiung Medical University (KMUH107-7R50, KMUH SA10807c), Kaohsiung Municipal Siaogang Hospital, Kaohsiung Medical University (H-108-005), and from Ministry of Science and Technology (MOST 108-2628-B-037-006), Taiwan.
Footnote
Conflicts of Interest: All authors have completed the ICMJE uniform disclosure form (available at http://dx.doi.org/10.21037/gs.2020.03.17). Tzu-Yen Huang: Dr. Huang reports grants from Kaohsiung Medical University Hospital, Taiwan, during the conduct of the study; Yi-Chu Lin: Dr. Lin has nothing to disclose; Hsin-Yi Tseng: Dr. Tseng has nothing to disclose; Gianlorenzo Dionigi: Dr. Dionigi has nothing to disclose; Hoon-Yub Kim: Dr. Kim has nothing to disclose; I-Cheng Lu: Dr. Lu has nothing to disclose; Pi-Ying Chang: Dr. Chang has nothing to disclose; Feng-Yu Chiang: Dr. Chiang has nothing to disclose; Che-Wei Wu: Dr. Wu reports grants from Kaohsiung Medical University; Hospital, Taiwan, grants from Kaohsiung Municipal Siaogang Hospital, Kaohsiung Medical University, Taiwan, grants from Ministry of Science and Technology, Taiwan, during the conduct of the study. GD has no conflicts of interest to declare.
Ethical Statement: The authors are accountable for all aspects of the work in ensuring that questions related to the accuracy or integrity of any part of the work are appropriately investigated and resolved. The animal-use protocol was consistent with national/international regulations and guidelines for animal experiments, including replacement, reduction, and refinement principles, and was approved by the Institutional Animal Care and Use Committee of Kaohsiung Medical University, Taiwan (IACUC protocol No. 108084).
Open Access Statement: This is an Open Access article distributed in accordance with the Creative Commons Attribution-NonCommercial-NoDerivs 4.0 International License (CC BY-NC-ND 4.0), which permits the non-commercial replication and distribution of the article with the strict proviso that no changes or edits are made and the original work is properly cited (including links to both the formal publication through the relevant DOI and the license). See: https://creativecommons.org/licenses/by-nc-nd/4.0/.
References
- Lang BH, Yih PC, Lo CY. A review of risk factors and timing for postoperative hematoma after thyroidectomy: is outpatient thyroidectomy really safe? World J Surg 2012;36:2497-502. [Crossref] [PubMed]
- Lachanas VA, Prokopakis EP, Mpenakis AA, et al. The use of Ligasure Vessel Sealing System in thyroid surgery. Otolaryngol Head Neck Surg 2005;132:487-9. [Crossref] [PubMed]
- Youssef T, Mahdy T, Farid M, et al. Thyroid surgery: use of the LigaSure Vessel Sealing System versus conventional knot tying. Int J Surg 2008;6:323-7. [Crossref] [PubMed]
- Melck AL, Wiseman SM. Harmonic scalpel compared to conventional hemostasis in thyroid surgery: a meta-analysis of randomized clinical trials. Int J Surg Oncol 2010;2010:396079. [Crossref] [PubMed]
- Papavramidis TS, Sapalidis K, Michalopoulos N, et al. UltraCision harmonic scalpel versus clamp-and-tie total thyroidectomy: a clinical trial. Head Neck 2010;32:723-7. [PubMed]
- Cannizzaro MA, Lo Bianco S, Borzi L, et al. The use of FOCUS Harmonic scalpel compared to conventional haemostasis (knot and tie ligation) for thyroid surgery: a prospective randomized study. Springerplus 2014;3:639. [Crossref] [PubMed]
- Duan YF, Xue W, Zhu F, et al. FOCUS harmonic scalpel compared to conventional hemostasis in open total thyroidectomy - a prospective randomized study. J Otolaryngol Head Neck Surg 2013;42:62. [Crossref] [PubMed]
- Agcaoglu O, Aliyev S, Mitchell J, et al. The use of the harmonic scalpel versus knot tying for modified radical neck dissection. Surg Innov 2013;20:81-5. [Crossref] [PubMed]
- Ferri E, Armato E, Spinato G, et al. Focus harmonic scalpel compared to conventional haemostasis in open total thyroidectomy: a prospective randomized trial. Int J Otolaryngol 2011;2011:357195. [Crossref] [PubMed]
- Yener O, Demir M, Yilmaz A, et al. Harmonic scalpel compared to conventional hemostasis in thyroid surgery. Indian J Surg 2014;76:66-9. [Crossref] [PubMed]
- Dionigi G, Boni L, Rausei S, et al. The safety of energy-based devices in open thyroidectomy: a prospective, randomised study comparing the LigaSure (LF1212) and the Harmonic(R) FOCUS. Langenbecks Arch Surg 2012;397:817-23. [Crossref] [PubMed]
- Dionigi G, Wu CW, Kim HY, et al. Severity of Recurrent Laryngeal Nerve Injuries in Thyroid Surgery. World J Surg 2016;40:1373-81. [Crossref] [PubMed]
- Wu CW, Lu IC, Randolph GW, et al. Investigation of optimal intensity and safety of electrical nerve stimulation during intraoperative neuromonitoring of the recurrent laryngeal nerve: a prospective porcine model. Head Neck 2010;32:1295-301. [Crossref] [PubMed]
- Randolph GW, Dralle H. International Intraoperative Monitoring Study Group, et al. Electrophysiologic recurrent laryngeal nerve monitoring during thyroid and parathyroid surgery: international standards guideline statement. Laryngoscope 2011;121 Suppl 1:S1-16. [Crossref] [PubMed]
- Dionigi G, Bacuzzi A, Boni L, et al. What is the learning curve for intraoperative neuromonitoring in thyroid surgery? Int J Surg 2008;6 Suppl 1:S7-12. [Crossref] [PubMed]
- Wu CW, Chai YJ, Dionigi G, et al. Recurrent laryngeal nerve safety parameters of the Harmonic Focus during thyroid surgery: Porcine model using continuous monitoring. Laryngoscope 2015;125:2838-45. [Crossref] [PubMed]
- Dionigi G, Chiang FY, Kim HY, et al. Safety of LigaSure in recurrent laryngeal nerve dissection-porcine model using continuous monitoring. Laryngoscope 2017;127:1724-9. [Crossref] [PubMed]
- Wu CW, Dionigi G, Sun H, et al. Intraoperative neuromonitoring for the early detection and prevention of RLN traction injury in thyroid surgery: a porcine model. Surgery 2014;155:329-39. [Crossref] [PubMed]
- Chiang FY, Lu IC, Kuo WR, et al. The mechanism of recurrent laryngeal nerve injury during thyroid surgery--the application of intraoperative neuromonitoring. Surgery 2008;143:743-9. [Crossref] [PubMed]
- Dionigi G, Donatini G, Boni L, et al. Continuous monitoring of the recurrent laryngeal nerve in thyroid surgery: a critical appraisal. Int J Surg 2013;11 Suppl 1:S44-6. [Crossref] [PubMed]
- Schneider R, Przybyl J, Hermann M, et al. A new anchor electrode design for continuous neuromonitoring of the recurrent laryngeal nerve by vagal nerve stimulations. Langenbecks Arch Surg 2009;394:903-10. [Crossref] [PubMed]
- Phelan E, Schneider R, Lorenz K, et al. Continuous vagal IONM prevents recurrent laryngeal nerve paralysis by revealing initial EMG changes of impending neuropraxic injury: a prospective, multicenter study. Laryngoscope 2014;124:1498-505. [Crossref] [PubMed]
- Lee HY, Cho YG, You JY, et al. Traction injury of the recurrent laryngeal nerve: Results of continuous intraoperative neuromonitoring in a swine model. Head Neck 2016;38:582-8. [Crossref] [PubMed]
- Wu CW, Huang TY, Chen HC, et al. Intra-Operative Neural Monitoring of Thyroid Surgery in a Porcine Model. J Vis Exp 2019. [Crossref] [PubMed]
- Lin YC, Dionigi G, Randolph GW, et al. Electrophysiologic monitoring correlates of recurrent laryngeal nerve heat thermal injury in a porcine model. Laryngoscope 2015;125:E283-90. [Crossref] [PubMed]
- Takada M, Ichihara T, Kuroda Y. Comparative study of electrothermal bipolar vessel sealer and ultrasonic coagulating shears in laparoscopic colectomy. Surg Endosc 2005;19:226-8. [Crossref] [PubMed]