Evaluation of skin viability in nipple sparing mastectomy (NSM)
Introduction
Nipple sparing mastectomy (NSM) and skin sparing mastectomy (SSM) have traditionally been avoided due to the inherent ischemia generated by the procedure and the resulting complications of skin and nipple loss. The standard of care has evolved to removal of the nipple-areolar complex (NAC) and the “at risk” skin and placement of a tissue expander in the subpectoral position. The advantages of this approach include the removal of potentially at risk tissues, a “delay” effect from gradual expansion and the avoidance of immediate pressure on the skin from a final implant placement. New technology and the experience of surgical pioneers have made it reasonable to reconsider NSM and immediate reconstruction of the breast with a final implant or tissue flap. This has been made possible by advances in our understanding of the vascularity of mastectomy flaps and technical improvements that unload pressure on the mastectomy skin, such as acellular dermal matrix (ADM) support of the implant. With unsurpassed reconstructive outcomes and high patient satisfaction, the procedure is positioned to become the new standard of care when oncologic considerations allow it.
The fundamental reason for early complications following NSM and SSM has been inadequate tissue perfusion in the mastectomy skin flaps (1-6). It follows then that reliable and accurate intraoperative evaluation of tissue perfusion would be helpful to limit complications and improve clinical outcomes. Clinical judgment is the most widely practiced method for flap evaluation (7), but by itself has not been completely reliable for perfusion assessment (8,9). Studies have shown that clinical assessment alone can be a poor predictor of postoperative complications (8-10). This can be further confounded by patient comorbidities, drugs, and previous treatments (Table 1). Ultimately, it is the variation in anatomic location of perforating vessels that supply the breast skin and the variation in the performance of mastectomy by breast surgeons that make NSM with immediate reconstruction a clinical challenge for so many. Any method to identify tissue at risk for necrosis would be a game changer for breast reconstructive surgeons. Many technologies to assess flap vascularity have been used clinically. Although some have been subjected to study, none have achieved universal acceptance (Table 2) (6,20).
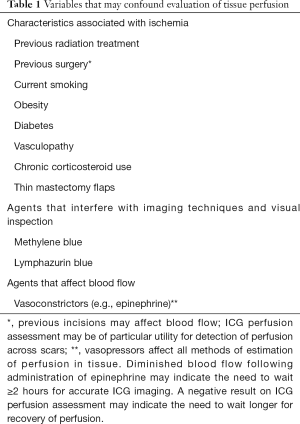
Full table
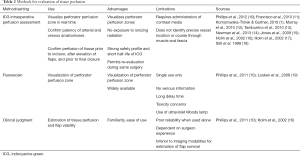
Full table
Modalities for the assessment of tissue perfusion
Surgeons generally rely on their judgment for flap evaluation, which generally involves subjective things like flap temperature, tissue color, capillary refill, and dermal bleeding. When clinical judgment is used alone however, even by experienced surgeons, outcomes can still be complicated because perfusion is hard to assess.
The Laser Doppler has been used for evaluation of blood flow within flaps. However, when used intraoperatively, it is highly variable in its readings. In the end, it can underestimate flap survival (21). The technique is also sensitive to small movements (21). A recent study reported that although laser Doppler was effective at identifying ischemia in free flaps, it had poor ability to detect perforator vessels (22). Although the technology continues have promise, it is not ready for standard use.
Transcutaneous oxygen (TCO2) measurement has been shown to improve flap salvage rates when used for postoperative monitoring (23,24). While some have brought TCO2 into the intraoperative setting, mapping flaps with this technology is time consuming and labor intensive. Initial findings suggest that it may have utility postoperatively as a continuous monitoring technology (25,26).
Fluorescein based angiography has also been used in the operating room to evaluate tissue perfusion. It requires the use of a Wood’s lamp. Fluorescein also has some limitations, include a long half-life, leakage from capillaries into the interstitium, and a clearance time of 12 to 18 hours. It is this long half-life which precludes re-evaluation during the intraoperative period, making it less useful (17,27-29). Furthermore, local ischemia enhances fluorescein diffusion, and this can lead to false-positive results (27,30). Head-to-head comparison suggests that fluorescein is not as accurate as ICG, with lower specificity and negative predictive value (10,16,17,19).
Indocyanine green (ICG) laser angiography (i.e., SPY Elite, SPYPhi)
ICG has been successfully used as a fluorescence imaging agent to evaluate perfusion in ophthalmologic (31,32), cardiac (33,34), vascular (35,36), and transplant surgery (37). Over the last decade, surgeons have used ICG angiography in plastic reconstructive surgical procedures and have demonstrated clinical utility (1,10-12,38). Since the ICG molecule binds strongly to plasma proteins, it remains in the intravascular space. It also has a short plasma half-life of 3 to 5 minutes in humans (39). These characteristics are important since the dye clears quickly from tissues, allowing repeated evaluations during the same surgical procedure. The dye is given intravenously and is excreted by the liver into the bile. Therefore, it is not associated with any nephrotoxicity. With ICG-based systems, a laser emits a near-infrared wavelength and accordingly does not require protective eyewear or other safety equipment.
Clinical and animal studies in reconstructive surgical procedures have reported strong correlation between the ICG data and postoperative outcomes (14,18,40-42). Both case series and prospective studies have shown ICG visualized intraoperative perfusion was associated with reduced rates of complications, specifically postoperative necrosis and flap loss. This has been observed in a variety of procedures, including implant surgery, pedicle flaps, and free tissue transfers (1,5,8,15-17,38,43,44).
Intraoperative perfusion assessment using ICG is a vascular imaging methodology that can be used in the intraoperative or postoperative setting to visually assess superficial blood flow. Characteristics of ICG angiography make it a desirable option for skin evaluation. The technique provides real-time assessment of tissue perfusion that has been correlated with clinical outcomes (1,5,6,10,38) and guides surgical decision making in many surgical disciplines. With mastectomy skin evaluation, early identification of insufficiently perfused tissue has helped guide intraoperative decision making, such as the need for intraoperative mastectomy skin resection or the decision to perform a surgical delay.
The information provided by ICG-based systems augment and strengthen clinical judgment and provides the surgeon with real-time visualization of tissue perfusion and vascular changes that result from surgical maneuvers. Additionally, the ICG is collected by the lymphatic system and ICG-based systems show promise in lymphatic surgery (sentinel lymph node sampling, lymphedema surgery).
Software aided evaluation of ICG fluorescence (i.e., SPY-Q™ Analysis Toolkit) provides additional analysis tools, including the measurement of peak fluorescence intensity, ICG ingress and ICG egress. The software also allows comparison of different areas within the tissues. These algorithms have been shown in Level 1 clinical study to accurately predict skin necrosis (see Figure 1). That said, the clinician remains responsible for determining at-risk tissue based on their standard clinical practices while the visual evaluation of perfusion images is additive.
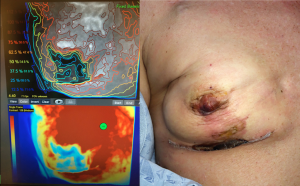
Nipple-sparing procedures
ICG-based systems can be used to evaluate perfusion in the NAC during mastectomies, including skin-sparing (SSM) and nipple-sparing mastectomies (NSM). Once the mastectomy has been completed, the NAC can be evaluated by examining both superficial epidermis and deep dermal and subcutaneous tissues to help determine whether the NAC is safe to use during the reconstruction. By examining the mastectomy skin and nipple with a sizer in place, ICG-based systems can help the surgeon decide to place the permanent implant or to delay the tissues and perform a staged reconstruction with placement of a tissue expander. There is also data to suggest that a preliminary scan prior to incision can show the dominance of blood supply to the NAC and help determine the best access incisions for the mastectomy (45).
Management of skin ischemia
Prevention is the key to the management of skin ischemia and prevention of necrosis a priority. When poor blood supply is suspected by clinical judgment and/or technologic means, direct-to-implant reconstruction should be temporarily abandoned and consideration given to delaying the procedure. Placement of a deflated tissue expander would be standard but the dissection necessary to place the expander below the muscle and the pressure of the device on the skin add unnecessary risk and may remove any chance of a nipple-sparing procedure. Additionally, placement of an expander commits the patient to months of expansion, possible nipple malposition due to the expansion process, and a second procedure to exchange the expander for a final implant. Completely backing out of the reconstruction and not placing an expander leaves open the possibility of a direct-to-implant reconstruction two weeks later. This “staged-immediate” procedure, also known as a “Zenn Delay” has been reported with excellent aesthetic results and a low complication rate (46). This technique offers certain advantages over the standard placement of a tissue expander (Table 3) may well represent the best approach for the breast reconstructive surgeon who desires to reconstruct NSMs but is experiencing skin or nipple loss in their practice (see Figure 2). In the setting of value-based care and depending on the incidence of necrosis and complications in a surgeons practice, use of the Zenn Delay would allow more patients to be successfully reconstructed at a lower cost to our healthcare system.
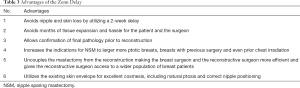
Full table
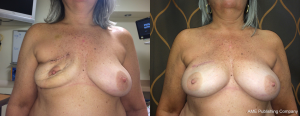
Conclusions
The performance of NSM is desired by our patients but inherently presents the risk of vascular compromise and complications. If one is experiencing such complications, technology can help delineate poor perfusion can provide a chance to intervene and prevent devastating complications. ICG-based systems have proven their clinical utility for the perioperative visualization of blood flow in mastectomy flaps. With the use of ADM now commonplace, this has allowed us to rethink immediate reconstruction with direct-to-implant or tissue reconstruction.
Acknowledgements
None.
Footnote
Conflicts of Interest: Dr. MR Zenn was Chief Medical Officer for Novadaq (SPY technology) 2016–2017, is a consultant for Stryker, and receives royalties from QMP (DVDs, app) and Thieme (textbook).
References
- Komorowska-Timek E, Gurtner GC. Intraoperative perfusion mapping with laser-assisted indocyanine green imaging can predict and prevent complications in immediate breast reconstruction. Plast Reconstr Surg 2010;125:1065-73. [Crossref] [PubMed]
- Sood M, Glat P. Potential of the SPY intraoperative perfusion assessment system to reduce ischemic complications in immediate postmastectomy breast reconstruction. Ann Surg Innov Res 2013;7:9. [Crossref] [PubMed]
- Garvey PB, Buchel EW, Pockaj BA, et al. DIEP and pedicled TRAM flaps: a comparison of outcomes. Plast Reconstr Surg 2006;117:1711-9; discussion 1720-1.
- Spear SL, Ducic I, Cuoco F, et al. The effect of smoking on flap and donor-site complications in pedicled TRAM breast reconstruction. Plast Reconstr Surg 2005;116:1873-80. [Crossref] [PubMed]
- Pestana IA, Coan B, Erdmann D, et al. Early experience with fluorescent angiography in free-tissue transfer reconstruction. Plast Reconstr Surg 2009;123:1239-44. [Crossref] [PubMed]
- Newman MI, Samson MC, Tamburrino JF, et al. An investigation of the application of laser-assisted indocyanine green fluorescent dye angiography in pedicle transverse rectus abdominus myocutaneous breast reconstruction. Can J Plast Surg 2011;19:e1-5. [Crossref] [PubMed]
- Zenn MR. Fluorescent angiography. Clin Plast Surg 2011;38:293-300. [Crossref] [PubMed]
- Munabi NC, Olorunnipa OB, Goltsman D, et al. The ability of intra-operative perfusion mapping with laser-assisted indocyanine green angiography to predict mastectomy flap necrosis in breast reconstruction: A prospective trial. J Plast Reconstr Aesthet Surg 2014;67:449-55. [Crossref] [PubMed]
- Kanuri A, Liu AS, Guo L. Whom Should We SPY? A Cost Analysis of Laser-Assisted Indocyanine Green Angiography in Prevention of Mastectomy Skin Flap Necrosis during Prosthesis-Based Breast Reconstruction. Plast Reconstr Surg 2014;133:448e-54e. [Crossref] [PubMed]
- Phillips BT, Lanier ST, Conkling N, et al. Can intraoperative perfusion techniques accurately predict mastectomy skin flap necrosis? Plast Reconstr Surg 2012;129:778e-88e. [Crossref] [PubMed]
- Francisco BS, Kerr-Valentic MA, Agarwal JP. Laser-assisted indocyanine green angiography and DIEP breast reconstruction. Plast Reconstr Surg 2010;125:116e-8e. [Crossref] [PubMed]
- Murray JD, Jones GE, Elwood ET, et al. Fluorescent intraoperative tissue angiography with indocyanine green: evaluation of nipple-areola vascularity during breast reduction surgery. Plast Reconstr Surg 2010;126:33e-4e. [Crossref] [PubMed]
- Tamburrino JF, Newman MI, Samson MC, et al. Laser-assisted indocyanine green fluorescent dye angiography (LA-ICG) in evaluation of mastectomy flaps. Presented at: 2010 Annual Meeting for AAHS, ASPN, and ASRM.: Boca Raton, Florida. 2010.
- Newman MI, Jack MC, Samson MC. SPY-Q analysis toolkit values potentially predict mastectomy flap necrosis. Ann Plast Surg 2013;70:595-8. [Crossref] [PubMed]
- Jones GE, Garcia CA, Murray J, et al. Fluorescent intraoperative tissue angiography for the evaluation of the viability of pedicled TRAM flaps. Plast Reconstr Surg 2009;124:53. [PubMed]
- Holm C, Tegeler J, Mayr M, et al. Monitoring free flaps using laser-induced fluorescence of indocyanine green: a preliminary experience. Microsurgery 2002;22:278-87. [Crossref] [PubMed]
- Holm C, Mayr M, Hofter E, et al. Intraoperative evaluation of skin-flap viability using laser-induced fluorescence of indocyanine green. Br J Plast Surg 2002;55:635-44. [Crossref] [PubMed]
- Still J, Law E, Dawson J, et al. Evaluation of the circulation of reconstructive flaps using laser-induced fluorescence of indocyanine green. Ann Plast Surg 1999;42:266-74. [Crossref] [PubMed]
- Losken A, Styblo TM, Schaefer TG, et al. The use of fluorescein dye as a predictor of mastectomy skin flap viability following autologous tissue reconstruction. Ann Plast Surg 2008;61:24-9. [Crossref] [PubMed]
- Tan BK, Newman MI, Swartz KA, et al. Subfascial perforator dissection for DIEP flap harvest. Plast Reconstr Surg 2009;124:1001-2; author reply 1002. [Crossref] [PubMed]
- Hedén P, Jurell G, Arnander C. Prediction of skin flap necrosis: a comparative study between laser Doppler flowmetry and fluorescein test in a rat model. Ann Plast Surg 1986;17:485-8. [Crossref] [PubMed]
- Schlosser S, Wirth R, Plock JA, et al. Application of a new laser Doppler imaging system in planning and monitoring of surgical flaps. J Biomed Opt 2010;15:036023. [Crossref] [PubMed]
- Steele MH. Three-year experience using near infrared spectroscopy tissue oximetry monitoring of free tissue transfers. Ann Plast Surg 2011;66:540-5. [Crossref] [PubMed]
- Lin SJ, Nguyen MD, Chen C, et al. Tissue oximetry monitoring in microsurgical breast reconstruction decreases flap loss and improves rate of flap salvage. Plast Reconstr Surg 2011;127:1080-5. [Crossref] [PubMed]
- Keller A. A new diagnostic algorithm for early prediction of vascular compromise in 208 microsurgical flaps using tissue oxygen saturation measurements. Ann Plast Surg 2009;62:538-43. [Crossref] [PubMed]
- Keller A. Noninvasive tissue oximetry for flap monitoring: an initial study. J Reconstr Microsurg 2007;23:189-97. [Crossref] [PubMed]
- Rübben A, Eren S, Krein R, et al. Infrared videoangiofluorography of the skin with indocyanine green--rat random cutaneous flap model and results in man. Microvasc Res 1994;47:240-51. [Crossref] [PubMed]
- Singer R, Lewis CM, Franklin JD, et al. Fluorescein test for prediction of flap viability during breast reconstructions. Plast Reconstr Surg 1978;61:371-5. [Crossref] [PubMed]
- Jones BM. Monitors for the cutaneous microcirculation. Plast Reconstr Surg 1984;73:843-50. [Crossref] [PubMed]
- May JW Jr, Chait LA, O'Brien BM, et al. The no-reflow phenomenon in experimental free flaps. Plast Reconstr Surg 1978;61:256-67. [Crossref] [PubMed]
- Dzurinko VL, Gurwood AS, Price JR. Intravenous and indocyanine green angiography. Optometry 2004;75:743-55. [Crossref] [PubMed]
- Flower RW, Hochheimer BF. A clinical technique and apparatus for simultaneous angiography of the separate retinal and choroidal circulations. Invest Ophthalmol 1973;12:248-61. [PubMed]
- Reuthebuch O, Haussler A, Genoni M, et al. Novadaq SPY: intraoperative quality assessment in off-pump coronary artery bypass grafting. Chest 2004;125:418-24. [Crossref] [PubMed]
- Desai ND, Miwa S, Kodama D, et al. A randomized comparison of intraoperative indocyanine green angiography and transit-time flow measurement to detect technical errors in coronary bypass grafts. J Thorac Cardiovasc Surg 2006;132:585-94. [Crossref] [PubMed]
- Kang Y, Lee J, Kwon K, et al. Application of novel dynamic optical imaging for evaluation of peripheral tissue perfusion. Int J Cardiol 2010;145:e99-101. [Crossref] [PubMed]
- Kang Y, Lee J, Kwon K, et al. Dynamic fluorescence imaging of indocyanine green for reliable and sensitive diagnosis of peripheral vascular insufficiency. Microvasc Res 2010;80:552-5. [Crossref] [PubMed]
- Sekijima M, Tojimbara T, Sato S, et al. An intraoperative fluorescent imaging system in organ transplantation. Transplant Proc 2004;36:2188-90. [Crossref] [PubMed]
- Newman MI, Samson MC. The application of laser-assisted indocyanine green fluorescent dye angiography in microsurgical breast reconstruction. J Reconstr Microsurg 2009;25:21-6. [Crossref] [PubMed]
- SPY Elite System. Kit Instructions for Use. Vancouver, Canada: Novadaq Corp., 2017.
- Duggal CS, Madni T, Losken A. An outcome analysis of intraoperative angiography for postmastectomy breast reconstruction. Aesthet Surg J 2014;34:61-5. [Crossref] [PubMed]
- Matsui A, Lee BT, Winer JH, et al. Predictive capability of near-infrared fluorescence angiography in submental perforator flap survival. Plast Reconstr Surg 2010;126:1518-27. [Crossref] [PubMed]
- Matsui A, Lee BT, Winer JH, et al. Quantitative assessment of perfusion and vascular compromise in perforator flaps using a near-infrared fluorescence-guided imaging system. Plast Reconstr Surg 2009;124:451-60. [Crossref] [PubMed]
- Chatterjee A, Krishnan NM, Van Vliet MM, et al. A comparison of free autologous breast reconstruction with and without the use of laser-assisted indocyanine green angiography: a cost-effectiveness analysis. Plast Reconstr Surg 2013;131:693e-701e. [Crossref] [PubMed]
- Yamaguchi S, De Lorenzi F, Petit JY, et al. The "perfusion map" of the unipedicled TRAM flap to reduce postoperative partial necrosis. Ann Plast Surg 2004;53:205-9. [Crossref] [PubMed]
- Gurtner GC, Jones GE, Neligan PC, et al. Intraoperative laser angiography using the SPY system: review of the literature and recommendations for use. Ann Surg Innov Res 2013;7:1-23. [Crossref] [PubMed]
- Zenn MR. Staged Immediate Breast Reconstruction. Plast Reconstr Surg 2015;135:976-9. [Crossref] [PubMed]