“Super-active surveillance”: MRI ultrasound fusion biopsy and ablation for less invasive management of prostate cancer
Introduction
Over one million prostate biopsies are performed in the United States with greater than 160,000 new diagnoses of prostate cancer every year (1,2). However, far fewer patients will go on to die of prostate cancer due to the often-indolent nature of this disease (3). More aggressive cancer requires definitive therapy in the form of radiation therapy (RT) or radical prostatectomy (RP), but these patients may suffer debilitating side effects. Men who undergo surgery are commonly at risk for erectile dysfunction (6–37%) and incontinence (4–31%) while patients opting for RT have these risks as well as the risk of severe long-term bladder or bowel dysfunction (1–5%) (4-6). Advances in prostate imaging with multiparametric magnetic resonance imaging (mpMRI) allow clinicians to visualize, characterize, and target specific lesions for biopsy or ablation. By more accurately sampling these lesions within the prostate, clinicians are less likely to miss clinically significant cancer and more likely to unveil the true grade of disease (7). With this technology, practitioners can more confidently select those who need treatment while simultaneously avoiding side effects and complications (7). Additionally, with mpMRI, clinicians not only have the ability to visualize lesions for biopsy, but also to plan local, partial/focal gland treatment. This has ushered in a new era in which focal therapy for prostate cancer is an emerging paradigm with a rapidly growing foundation. These therapies have been developed in an attempt to stop or delay the effects of prostate cancer, while sparing the entirety of the gland (and adjacent structures) so that the patient may maintain his quality of life.
Prostate cancer has historically been detected by random systematic biopsies (Sbx) and the risk of under-sampling the cancer with Sbx nears 30% in many studies, with the chance of missing cancer altogether nearly the same (8-11). Those with low-grade cancer on systematic biopsy are at even higher risk of upgrading when compared to final pathology, which often creates worry in the minds of physicians and patients choosing active surveillance as a management option (10,12). These errors are in large part due to under-sampling and the inability to adequately visualize or sample difficult-to-reach areas of the prostate, such as the anterior or apical portions of the gland (9). Prostate mpMRI allows visualization of lesions within the prostate with subsequent fusion biopsy better representing the true cancer volume and grade (7,13-15). Prostate mpMRI has a reported sensitivity of 44–87% with a negative predictive value of 92–94% (16). However, small tumors, <0.5 mL, are often difficult to visualize and may still present a diagnostic challenge (17). Additionally, mpMRI may underestimate lesion size, reportedly up to as high as an average of 11mm in diameter (18), although the underestimation error may be more pronounced with low field 1.5T MRI without endorectal coil (ERC). Despite these limitations, biopsies targeted by MRI information yield more accurate results. Siddiqui et al. (7) reported that 30% more high-grade cancers were found with fusion biopsy, along with a 17% decrease in low-grade cancers (that include clinically insignificant cancers that may not need whole-gland treatment at all).
In-gantry MRI biopsy and cognitive fusion biopsy
In-gantry real-time MRI may aid prostate biopsy via a transrectal or transperineal approach (19). Some proponents still advocate for in-gantry MRI targeted biopsy, claiming it is more accurate than transrectal ultrasound (TRUS)/MRI fusion, without misregistration error. However, custom equipment is required, fewer samples in general take longer to obtain, and the supply of available MRI, skilled physicians, and cost-effective resources may not be widely available for such diagnostic interventional procedures. The search for a faster and easier method to use the MRI information outside of the gantry led to the development of TRUS/MRI fusion systems and techniques. These are more accessible to practitioners and patients, and are easier and potentially less costly. The simplest and likely least accurate method is the “cognitive fusion” technique, where the practitioner visualizes the mpMRI separately and then visualizes the location with real-time ultrasound prior to performing the TRUS guided biopsy. The disadvantage of this technique is that the results depend on the experience of the practitioner and small lesions may be harder to appropriately sample (20). MRI and ultrasound imaging planes are in differing orientations and angles of splaying or fanning, which complicates base to apex specificity while correlating MRI to end-fire axial or side-fire sagittal TRUS. The main advantage of this less accurate cognitive method is the cost savings, since no additional equipment or software is required. When performed by experienced clinicians, cognitive fusion biopsy cancer detection rates have been reported comparable to methods that computationally fuse the mpMRI and ultrasound images (14). However, fusion devices are likely more accurate, more reproducible, more quickly overcome the learning curve, miss fewer secondary histological findings (14,20), and likely better score and characterize patients’ cancers, when correlated directly to prostatectomy specimens. It is proven that cognitive fusion could improperly and inaccurately characterize target tumors, which may be more pronounced in less experienced operators (21).
Workflow for fusion biopsy
In order to take advantage of better quality mpMRI images, new software, devices, and platforms have been developed to fuse the mpMRI images with the real-time ultrasound image for target detection during biopsy. This does not require the physical presence of the MRI gantry during biopsy. A diagnostic MRI is performed and the prostate gland edge is segmented (the edge is outlined) manually or semi-automatically, and the target tumor is either outlined, or its center-point is annotated. The segmentation (outline) and tumor (target) are usually sent to the fusion biopsy system via a picture archiving and communication system (PACS) workstation, or intranet network. The plan is made and reviewed, including mapping the location and 3D coordinates of any prior positive targets for patients on active surveillance.
The prostate ultrasound images are taken by sweeping or acquiring views in different axes. Early in the procedure, the mpMRI and ultrasound images must undergo a rigid or elastic registration which aligns the images for morphology and scale to take into account the deformation by the ultrasound probe, ERC balloon, prostate motion and deformations, and movements by the patient. Elastic image fusion may be chosen to try to preserve the matching to account for real-time changes of the gland while the patient undergoes the procedure (20). This requires warping the MRI to match the ultrasound such as with the UroNav® (Invivo, Gainesville, FL, USA), or warping the ultrasound to match the MRI such as with Trinity® (Koelis, Meylan, France). The key feature of the fusion biopsy is the tracking and registration of the ultrasound (using electromagnetic tracking, position sensing with encoded joints, image based tracking, or transducer gyroscopes) so that the system continually knows the location the probe is visualizing and targeting. Different platforms have accomplished this through different means. One method of tracking involves placing an electromagnetic field near the patient. This is employed by devices such as UroNav®, Real-Time Virtual Sonography (RVS) (Hitachi, Tokyo, Japan), Virtual Navigator® (Esaote, Florence, Italy), and BK Fusion® (Analogic, Peabody, MA, USA) (20,22-25). Alternatively, the Artemis System® (Eigen, Grass Valley, CA, USA), Biojet® (DK Technologies, Barum, Germany), Fusion Bx (Focal Healthcare, Toronto, Canada) and Biopsee® (Oncology Systems Limited, Shropshire, England) use a different registration technique that incorporates a mechanical arm with encoded joints attached to the ultrasound probe to report movement and feedback to the system (20,26-29). One last modality to register location is real-time ultrasound feedback which uses the initial ultrasound scan to re-create the prostate 3D model and track the needle based solely on ultrasound images (20,28). This technology is employed by Trinity®. Another difference between these systems is the ability to perform biopsies transperineally or transrectally. All of these systems have been developed to be used both transperineally and transrectally with the exception of the Biopsee® which is currently made for transperineal use only (23-26,29-32). With these systems, targeted prostate biopsies can be performed easily and reliably to accurately characterize and hunt for high-risk disease. Besides improved detection of high grade cancer, fusion prostate biopsies are useful for guidance of minimally invasive image-guided treatment modalities. Fusion biopsies and mpMRI can map and record previous biopsy sites for future mapping and use, and improve monitoring of cancer progression for patients on active surveillance (33,34). Furthermore, with mpMRI and targeted biopsy results, lesions within the prostate can be targeted for ablation, including sites of mapped disease from random sextant biopsies.
Ablative techniques
As imaging for diagnosis evolves using MRI or TRUS/MRI fusion, the more reliable characterization of tumors leads to more comfort with active surveillance as well as a “super-active surveillance” or focal therapy.
Focal therapy is defined as the specific targeting and ablation of the malignant portion of the prostate while leaving benign tissue intact. Multiple energy modalities have been used to ablate target cancer tissue such as cryoablation, high-intensity focused ultrasound (HIFU), radiofrequency ablation (RFA), laser ablation, irreversible electroporation (IRE), microwave ablation, photodynamic therapy and convective water vapor. Generally, the index lesion (biggest and highest-grade Gleason lesion) is among the main areas targeted, but depending on the type of energy used and quadrant affected, hemi-gland or whole gland ablation procedures have been employed (35), as well as multiple scattered lesion focal ablation which is more common with focal laser ablation (FLA) than with cryoablation.
The ideal patient for focal therapy is still debated, without consensus or validated long-term data. Initial experience with this technology began with low risk patients who now are known to be more suited for active surveillance. More recently, the focus may have shifted to treating patients with slightly more aggressive disease such as 3+4 Gleason (35,36). Currently, based on one European attempt to form consensus, patients with intermediate-risk disease (PSA 10–20, Gleason Score (GS) 3+4 or 4+3, or clinical stage T2b–T2c) as classified by the National Comprehensive Cancer Network (NCCN) and with a life expectancy ≥10 years are most likely to benefit most from this therapy (35,37). Stricter criteria include number, length, and location of positive biopsy cores, as well. Truesdale et al. validated consideration of these additional measures by demonstrating that total biopsy tumor length and number of positive biopsy cores are variables significantly associated with biochemical disease progression (38). This rate of progression is highly dependent upon age and length of follow up for such a slow-growing disease. One opinion (however without specific consensus, accepted criteria, or high level long term evidence) might be that many patients who are candidates for active surveillance may also be candidates for ablation therapies (we sometimes call “super-active surveillance”). Certainly, features such as extent of disease or likelihood for progression should influence selection criteria. Physicians who believe that prostate cancer is a multifocal disease may recommend whole gland or nearly-whole gland therapies over focal ablation. As you can ascertain from the nebulous nature of this discussion, this field is a “work in progress” regarding exact indications and long-term expectations. Focal ablation with fusion guidance is best performed under an investigational study, such that the broad community can address some of these lingering questions, which can only be answered by hypothesis-driven long-term clinical trials.
The main criticism against focal ablation is that prostate cancer is commonly a multifocal disease in up to 90% of cases, and the approximate rate of having only unilateral disease is generally accepted to be between 20–30% (39-41). Therefore, any ablative technique will miss significant amounts of cancer, which may risk progression or metachronous recurrence of otherwise curable disease. Alternatively, there is evidence that the index lesion in certain patients is the driver behind future cancer progression, and that other lesions within the prostate could represent insignificant cancer (39,40). These secondary lesions are often low volume and Gleason 3+3. One study found that secondary cancers had a cumulative volume of 0.3 cm3, while another found these lesions to be <0.5 cm3 (39,42). These lesions are similar to those detected incidentally in cystoprostatectomy specimens or found incidentally on autopsy (43,44). Furthermore, in a RP cohort, these secondary lesions had no influence on the rate of biochemical recurrence (BCR) as compared to the primary lesion (45). Contrary to these insignificant tumor foci, the index lesions are the largest, often with highest GS and with the potential to penetrate the capsule and/or metastasize (46,47). Therefore, while prostate cancer is almost always multifocal, the metastatic potential is often derived from a focus of tumor cells of monoclonal origin (48), often contained within an index lesion. The supported hypothesis is that destruction of this focus may be the only necessary treatment to prevent metastatic disease in certain patients.
Focal ablation has the potential to treat prostate lesions detected on mpMRI while sparing patients the devastating consequences of RP or RT. These techniques have garnered renewed interest due to advances in mpMRI, which as a partner to ablation, boosts the value of focal or sub-total gland ablation therapies. In these patients, MRI is theoretically able to detect and raise a red flag for enlarging lesions, or those with altered characteristics. Specific MRI criteria for post-ablation analysis are still being actively developed and validated. While prostate cancer is almost always a multifocal disease, the significance of the non-dominant lesions is questionable, making focal therapy a real possibility for certain highly selected patients. We describe the existing techniques of prostate focal ablation which are often performed as an overnight, ambulatory or even office-based procedure. However, some patients with severe benign prostatic hypertrophy (BPH) or comorbidities may require longer term admission, or a home catheter until swelling subsides, to avoid urinary retention.
Cryotherapy
Cryotherapy is a thermoablative technology designed to target malignant foci with sub-freezing temperatures (−40 °C), while preserving the functionality of adjacent, non-cancerous structures. To date, cryotherapy has shown to be a minimally morbid procedure. However, despite restrictive inclusion criteria, long term oncologic control remains widely variable, although very high success rates have been reported (49,50).
Delivery and expansion of inert gases within the prostatic tissue generate microenvironments with temperatures sufficient for cryoablation (51). Freezing results in cell membrane disruption, cellular edema, apoptosis and coagulative necrosis several millimeters inside the cryolesion, or “ice ball” (52). To selectively freeze the prostate, argon/helium or nitrogen-based cryoprobes are inserted via a transperineal approach. Modified brachytherapy grids with transperineal steppers aid in directing the probes to the desired prostate region, and TRUS is used for in-vivo visual monitoring of probe placement and cryolesion generation (51,53). To increase the accuracy of cryolesion placement, software platforms assist in pre-procedure planning and intraoperative image guidance. TRUS-guidance is the traditional method, but the cryolesion ice shadows ultrasound imaging, prompting investigation of magnetic resonance-guided platforms (54).
Studies indicate that lethal cryoablation of cancer cells is achieved at temperatures ≤−40 °C (55-57). Current cryotherapy systems utilize the Joules Thompson effect, in which argon gas is compressed for a rapid freezing effect and helium gas is used for thawing. As the gasses undergo phase transitions, energy is expended from the tissues and freezing results. As was first reported by Onik et al., a series of two consecutive freeze-thaw cycles more effectively cryoablates tissue (53,56). Factors such as freeze-thaw velocity, number of cycles, vascularity, and temperature nadir affect the cryoprobe’s capacity for cellular destruction (56). In a comparative study of cryotherapy versus low-dose brachytherapy, Gestaut et al. suggested cryotherapy may be less efficacious than when using a freezing goal of −35 °C as compared to −40 °C (58). To monitor the freezing (or heating) effect on the prostate and adjacent structures, temperature probes may be placed at various locations throughout the prostate including the cryoprobe location (to determine production of sufficient cryoablative temperatures), and at sites vulnerable to freezing-related complications, such as the neurovascular bundles, rectal mucosa, and striated external sphincter. Collateral cellular damage is tissue dependent and can occur at −15 °C or higher, and neurovascular dysfunction can occur at 3 °C, well above the desired temperature for focal cryotherapy (59). While it is reported that temperatures decrease to −40 °C for effective freezing within 5 mm of the leading edge of the cryoprobe, the periphery of the ice ball is 0 °C (55,60). This is insufficient for cancer cell death, but can still cause harm to adjacent thermally-sensitive vital structures. Preclinical studies propose extending the cryolesion perimeter 10 mm beyond the indicated lesion to increase the likelihood of exposing the entire tumor to −40 °C temperature, but this practice could pose risk for complications and long-term morbidity (60).
Reported rates of urinary incontinence and erectile dysfunction are fairly low ranging from 0–4% and 10–39%, respectively, as compared to 6–37% and 4–31% for robotic RP (4-6), but patient selection bias varies widely, there is no direct comparison quality data available, and the definitions and chronicity of complications vary widely. In studies conducted by Truesdale et al. and Valerio et al., post-therapy urinary symptom scores were improved when compared to pre-therapy results (38,54). More severe reported complications include rectourethral fistula, perineal fistula, necrosis of corpus cavernosum, abscess, urethral stricture, and urinary retention (61). However, these complications are infrequent and as reported by Durand et al. may resolve with minor additional intervention (61). To mitigate potential complications from localized freezing, a specialized urethral warming catheter is used and normal saline (NS) is injected into the potential space between Denonvilier’s fascia and the rectum to hydrodissect and protect these structures (50). Another protective technique is done for FLA, in which a chilled urethral catheter is used along with NS hydrodissection. Dextrose 5% in water hydrodissection is not recommended due to massive sodium shift leading to hyponatremia with large volume hydrodissections. In most studies, the procedure is done in the outpatient setting and the patient is discharged with a Foley catheter for 3–10 days, although the majority of focal ablation patients at our institution had catheters removed on the day of, or one day after the procedure. History of retention post-biopsy should warrant a conservative approach.
As seen in Table 1, follow-up frequency, duration, and methods vary widely among studies, but most use BCR as the primary endpoint to determine biochemical disease-free survival (BDFS). There are currently no universal criteria for BCR. The two most-often used are the American Society for Radiation Oncology (ASTRO) criteria, (which defines BCR as three consecutive rises in PSA beginning at least 6 months after focal therapy), and the Phoenix criteria, (which defines BCR as an increase in PSA of 2 ng/mL above the nadir). However, whether focal or hemi-ablative cryotherapy is performed, viable prostate continue to produce PSA. PSA kinetics in a partially ablated prostate are different than those following whole-gland ablation or RP, in which PSA should be undetectable (57). Marked decreases in post-therapy PSA are seen in multiple studies, but as Bahn et al. demonstrated, rises in post-therapy PSA are not consistent indicators of BCR (56). In attempting to move away from serial PSA-based criteria, Valerio et al. also employed mpMRI to detect recurrent cancer foci (54). In seven peer-reviewed clinical studies, patients underwent repeat prostate biopsies for BCR or routinely at 1-year post-therapy. For those routinely re-biopsied (independent of PSA trends), rates of positive cores on repeat biopsy ranged from 0–26% (50,56,57,61). For studies in which re-biopsy occurred after BCR per PSA-based criteria (e.g., ASTRO, Phoenix), rates of positive cores ranged from 8–45% (38,51,62). If one believes in the “index lesion theory” previously described, then the significance of a Gleason 6 recurrence is uncertain, often depending upon age.
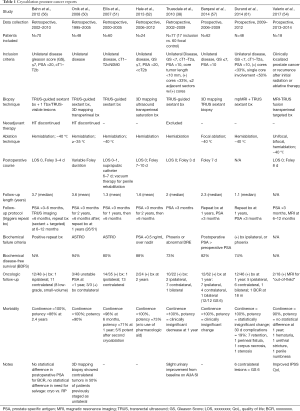
Full table
After pooling study results, 16 (25%) positive cores were sampled from the previously cryoablated region (ipsilateral) of the prostate, 44 (70%) were from the contralateral prostate, and three (5%) were found bilaterally. In the majority of cases, the positive cores were Gleason score 6 disease (38,50,51,56,57,61,62). Of the positive repeat TRUS biopsies, Bahn et al. were unable to visualize lesions in nine of 11 (56). Overall reported rates of BCR-free survival ranged from 73–94%. The use of PSA or scheduled repeat biopsy to determine treatment failure did not appear to alter BCR (38,50,51,57,61,62).
For patients with cancer detected on repeat biopsy, many elected for additional cryoablations or active surveillance (38,50,51,56,57,61,62). Reported morbidity from repeat cryotherapy appears minimal. For example, in a study conducted by Ellis et al., each of the five patients who underwent a second round of cryotherapy remained potent (51). Furthermore, in a matched pair comparison of focal cryotherapy and RP, Bahn et al. found a similar need for salvage therapy between these two primary treatment modalities (56). Figure 1 shows an example of patient with residual cancer following cryoablation.
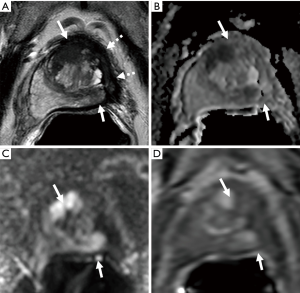
Focal cryoablation is the most studied modality for selectively treating prostate cancer. To date, clinical studies of focal therapy have successfully demonstrated a low rate of associated morbidities (most notably incontinence and impotence), when targeting unilateral, low risk prostate cancer. Use of multiple temperature sensors has mitigated risk of damaging adjacent structures in order to maintain function. Determination and definition of focal cryotherapy’s efficacy remains a significant challenge. PSA-driven criteria for BCR, while ubiquitous, are inherently flawed. Identifying patients for re-biopsy based on PSA measurements may miss a significant number of patients with recurrent prostate cancer, while oversampling the majority of BCR patients exposes them to unnecessary risks. Use of repeat imaging may offer better follow-up strategies as mpMRI will distinguish high- from low-grade indolent lesions (7). However, the relationship between postoperative changes and progression of disease in ablative patients is not well understood. Like other forms of focal therapy, focal cryotherapy may be a viable option for patients with low-risk, low-volume prostate cancer, who prefer intervention over active management or “super-active surveillance” over active surveillance.
HIFU
Use of ultrasound waves to destroy living tissue dates back at least to 1944 (63). Due to this destructive capability, HIFU has been evaluated for the treatment of a wide array of solid tumors (64). The principle behind HIFU is that electrical current is applied to piezoelectric crystals, which generate a field of ultrasound waves emanating from the instrument’s transducer (64). These fields are focused to induce mechanical, thermal, or radiation force changes to destroy or rupture cells. Absorbed acoustic energy is focused and converted to heat, with variable degrees of inertial and non-inertial cavitation possible. Cavitation is the sometimes violent implosion of gas at one focus (64).
HIFU to destroy prostate tissue was described in 1993 to treat benign prostatic hyperplasia (BPH) (65). Since then, HIFU has been applied broadly for treatment of BPH, and to a lesser extent prostate cancer, with whole-gland ablations, hemi-ablations, and now focal therapy (66). It has been a treatment option for patients with prostate cancer since at least 2004 in Europe, and in 2015 was approved by the Food and Drug Administration (FDA) for ablation of prostate tissue in the United States (66). The procedure lasts between one to three hours, depending on prostate volume, can be done as an outpatient under general or spinal anesthesia, and requires the use of either a Foley or suprapubic catheter afterwards (67). The ideal gland size for HIFU is 40 mL or less without calcifications, which cause disruption in ultrasound wave transmission (67). Larger volumes can be considered, but may require longer operative times, trans-urethral resection of the prostate (TURP), neoadjuvant androgen deprivation, or other pharmacotherapies (67).
Like many other ablative technologies, the definition of treatment failure is not clearly or uniformly reported in the literature. In a retrospective study of 285 patients with low risk (LR) or intermediate risk (IR) disease (68) and a median follow up of 4.7 years, 71 (24.9%) patients failed treatment as defined by the need for further treatment, additional cancer found on biopsy, prostate cancer metastases or death. These authors found that PSA nadir +1.2 ng/mL which they termed the “Stuttgart criteria”, was a better predictor of failure than other criteria such as the ASTRO or Phoenix criteria (68). Figure 2 shows residual disease in a patient who underwent HIFU.
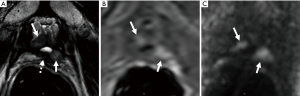
HIFU reports for safety and efficacy rates vary widely. HIFU’s safety was originally established in larger prospective trials beginning in the late 1990s for whole-gland ablation (69) or treatment for BPH. Whole gland ablation involves ablating as much tissue as possible, while attempting to leave the neurovascular bundles and urinary sphincter intact. In a study by Pinthus et al. (70), of 402 patients (45.5% LR and 54.5% IR) who had received whole gland HIFU, the mean PSA nadir after treatment was 0.36 ng/mL at 3 months. By Stuttgart criteria the BCR-free rates for LR and IR were 72% and 68%, respectively, at 4 years (70). Blana et al. (68) studied 285 patients who underwent whole gland ablation of whom 71 (25%) failed according to their criteria of a repeat positive biopsy or requiring salvage therapy. In this cohort, the median PSA nadir was 0.13 ng/mL which occurred at 12.9 weeks after therapy (68). One of the largest series of whole gland ablation by Crouzet et al. (71) recruited 1,002 patients (35.6% LR, 45.1% IR, 17.4% HR disease) with a median 6.4-year follow up. The authors report their 5- and 8-year BCR-free rate for LR (86% and 76%), IR (78% and 63%) and high-risk (HR) (68% and 57%) patients (71). The overall metastases free survival in this group was 94% (71). A summary of these studies can be seen in Table 2.
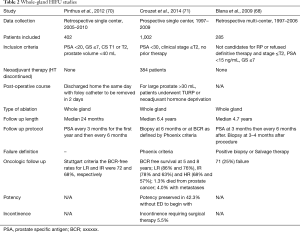
Full table
After whole-gland ablation established the safety of HIFU in the treatment of localized prostate cancer, recent studies as seen in Table 3, have focused more on hemi-ablative and focal therapy. Rischman et al. (72) suggests that hemi-ablation be used to extend treatment margins, compensate for the limitations of current imaging, and create a more reproducible procedure. Results are summarized here but vary widely. A prospective trial by van Velthoven et al. (73) of 50 patients who underwent HIFU hemi-ablation for LR or IR cancer and were followed for an average time of 40 months demonstrated 100% cancer-specific survival (CSS) and 93% metastases-free survival (MFS) (73). The PSA nadir was achieved in approximately 3 months following the procedure with a reduction of 72% from pre-treatment PSA (73).A prospective multicenter trial (72) examined 111 (68% LR and 32% IR) patients who underwent hemi-ablation HIFU monitored disease progression with PSA and mpMRI guided targeted biopsy at 6–12 months. It was found that 95% did not have any cancer detected in limited focal biopsy on the ablated side (72). Another prospective study by Feijoo et al. (74) of 71, mainly LR, patients undergoing hemi-ablation, used Phoenix criteria to determine BCR and found that 9.7% of patients recurred with 14.7% having a positive biopsy on the side of ablation at 1 year. A summary of partial and hemi-ablative studies can be seen in Table 4. El Fegoun et al. (75) reported on 10-year outcomes of 12 patients who underwent focal ablation. Only one patient in this group had a positive biopsy at 1 year (75). Additional studies have examined multifocal ablative techniques. A prospective trial included 42 men in their multifocal cohort undergoing treatment for multifocal lesions with HIFU (80). Of these patients, 39 men underwent a biopsy 6 months after treatment and nine (23%) had cancer, with 3 (8%) of those being clinically significant cancer (80). Five chose active surveillance and 4 chose retreatment (80). The reported that in men with good baseline functions, 84% at 1 year were continent, able to have sexual intercourse and had no evidence of clinically significant cancer (80). A similar study with included 52 men with treatment of only the lesions (81). At their 6-month biopsy, 57.7% had benign biopsies, while 80.1% were free of clinically significant prostate cancer. Of the 41 patients with good baseline characteristics, 53.7% at 1 year were continent, able to have sexual intercourse and had no evidence of clinically significant cancer (81).
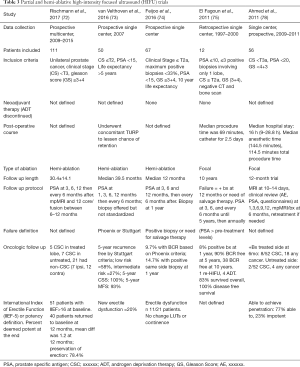
Full table
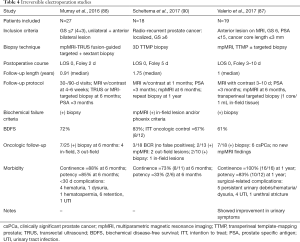
Full table
Erectile function after HIFU, in those patients with good function prior to undergoing focal or hemi-ablation, has been reported to be greater than 75% (66,72-74,76). Erectile dysfunction (ED) after HIFU likely depends on the degree and location of ablation in terms of proximity to the neurovascular bundles. In a prospective study by Ahmed et al. (76) those who underwent hemi-ablation by HIFU, 95% were able to have intercourse at 12 months post-op as compared to 40% in their series who underwent whole-gland ablation (82). Rischmann et al. (72) report on HIFU complications in 111 patients at a mean of 30.4±14.1 months. Of the patients who could achieve an erection before HIFU, 78.4% of patients had preserved erectile function at 12 months (72). Additionally, 97.2% of patients were totally continent (72). Other studies report low risks of incontinence after HIFU ranging from 5–12% (71,83). In a small cohort of 12 men, El Fegoun et al. (75) reported on complications at 10 years. All patients were pad-free at 10 years, but erectile function was not reported because many patients had underlying ED prior to therapy (75). Other reported complications included lower urinary tract symptoms (LUTS), urinary retention, urinary tract infection (UTI) and urethral stricture and fistula (73,84).
HIFU has also been employed as a salvage focal therapy for those patients who have failed RT. Hussein et al. (84) described 39 patients who underwent salvage HIFU ablation. Although these patients did have worsening incontinence (12.8%) and erectile function, of those who experienced a PSA nadir the BCR-free rate at 1, 2 and 3 years were 86%, 75% and 63%, respectively based on Phoenix criteria (84). Another study by Baco et al. (85), included 48 patients with BCR after RT. Again, worsening incontinence and erectile function were noted but the overall progression free survival (PFS) at 12, 18, and 24 months were 83%, 64%, and 52% respectively (85). As with many focal therapies, the results are likely institution, operator, and patient-dependent. All results from retrospective reports without control arms in a slow growing disease such as this need to be viewed with circumspection.
IRE
IRE utilizes high voltage electrical currents across a precisely defined intra-prostatic field to cause membrane pores and cell destruction. Unlike other forms of focal therapy for prostate cancer, IRE does not rely upon thermal mechanisms for its mode of action. Instead, the generated electrical currents create nanopores in cell membranes resulting in irreversible cell instability (86). A proposed advantage of a mainly non-thermal modality is theoretically less convective heat dissipation, which may avoid heat sink, energy under-delivery and therefore, under-treatment. In addition, pre-clinical models suggest that structures with high collagen content or tubular geometry, or long cells, (e.g., neurovascular bundles, muscle) more rapidly recover from IRE than prostatic tissue (87,88). In terms of this possible strength, exact implications for this technology have yet to be fully defined and verified.
Following pretreatment imaging [currently performed with mpMRI or transperineal template mapping prostate biopsy (TTMP)], IRE is conducted with transperineal placement of probes under ultrasound guidance to establish an ablative field (77,79,89,90). Presently, the NanoKnife platform (Angiodynamics, Queensbury, NY), is the only FDA 501(k) cleared IRE system, although it has limited clinical use thus far. Once in place, initial pulses determine the appropriate current generated between probe pairs, and then additional pulses are generated for ablation. For efficacious ablation, early clinical studies recommend a maximum distance between probes of 20 mm and at least 5 mm separation from the prostatic capsule (77,89).
The use of electric current necessitates the patient receive general anesthesia and full muscle paralysis to prevent aberrant muscle contractions or arrhythmias. Fortunately, operative time is relatively short. In a pilot study conducted by Murray et al., the median ablation time was 14 minutes, and similarly, in a study by Valerio et al., median surgery time was 64 minutes and median anesthesia time was 95 minutes; all patients were discharged in less than 24 hours (77,79).
In a number of clinical studies (Table 4) follow-up consisted of an mpMRI with contrast 1–6 weeks after IRE, mpMRI at 6 months, and repeat biopsy at 6 months to 1 year. PSA was assayed at 3-month intervals (77,79,90). Occasionally, contrast-enhanced ultrasound (CEUS) was used for pre-treatment and post-treatment imaging (91,92). Follow-up imaging is used to confirm the area of ablation and to detect new or recurrent lesions. By creating three-dimensional volumetric models generated from mpMRI, CEUS, and histopathology, van den Bos et al. (93) determined mpMRI and CEUS to be accurate measures of ablation zone volumes, although both modalities slightly overestimated the ablation volume as compared to histopathology.
Treatment complications were similar to complications seen in most transperineal procedures. Hematuria was most common. Dysuria, urinary retention, and UTIs were also seen (77,79,89). In three clinical studies evaluating the use of IRE in primary and salvage scenarios, post-treatment continence rates ranged from 73–100% and potency rates ranged from 33–85% (77,79,90). The salvage IRE trial yielded the lowest results for continence and potency (90). With median follow-up between 0.9–1.8 years, oncologic control data is limited. Percentage of patients without a positive follow-up biopsy core ranged from 61–72% (77,79,90).
IRE is a relatively new option for focal therapy of localized prostate cancer and its emergence as a predominantly less-thermal ablative technology makes it attractive for further investigation. Early studies demonstrate low morbidities including continence and potency rates comparable to other focal ablative therapies. It also has shown to be a viable outpatient procedure, but need for general anesthesia and paralytics incurs additional risks and narrows the potential patient population and clinical utility. In its nascent stage, IRE has yet to demonstrate significant short or long term oncologic efficacy, but it may also be used in conjunction with advanced imaging and biopsy platforms. If IRE truly eventually proves to spare nerve damage in a clinically relevant fashion, it may have theoretical advantages, although this is speculative at present and experience is limited.
FLA
The application of laser technology has become widespread in the medical community and costs have substantially decreased over time. Laser energy has been utilized in the urology community in applications such as prostatic tissue vaporization for BPH and urolithiasis lithotripsy. Prostate tissue is ideal for laser ablation due to a relative dearth of intrinsic vascularity compared to other organs (such as the liver and kidney) as well as its optical absorption rate (94). FLA allows for homogenous coagulative tissue necrosis. Prostate FLA has been historically used via MRI guidance, due to superior targeting accuracy vs. ultrasound-only techniques, and intrinsic MRI-compatibility of optical fibers. Using a specialized grid, fibers are introduced into the prostatic tumor target via a transperineal approach. This approach may also allow for greater accuracy in targeting lesions, especially those in the apical region. Additionally, targeting adjustments can be made in real time under imaging guidance. Saline displacement of heat-sensitive anatomy like rectum, urethra and bladder cooling via bladder catheter irrigation, and temperature monitoring are employed to ensure an appropriate temperature is reached to destroy the focus of cancer, while attempting to minimize any damage to surrounding structures. There appears to be a predictable linear increase in tissue temperature with longer laser use, as well as with an increased intensity and exponentially decreasing temperature with tissue depth (95). This translates into sharp transition zones between treated and untreated (thus uninjured). The sharp transition margin has become a differentiating feature for many operators, who value this laser chisel tool for sculpting away small focal prostate tumors. The laser originally used for ablative techniques was the 1,064 nm Nd:YAG laser but the diode (980 or 1,064 nm fiber) lasers now used have been found to be readily available, powerful, and cost-efficient (96).
An initial pre-clinical study used MRI-guided FLA with real-time monitoring in seven canines with prostate tumors. The laser applicator was able to be placed within an average of 1.1±0.7 mm of the target site, revealing a high degree of accuracy (97). When trialed in humans, Lee et al. (96), performed the procedure by typically giving sedation or general anesthesia, while the entire procedure was performed in the MRI suite. A standard ultrasound or MRI-guided peri-prostatic block should be performed for local anesthesia. FLA can be performed trans-rectally or transperineally. For the transrectal approach in MRI, a needle sleeve biopsy guide is placed into the rectum and the localization apparatus is attached to this biopsy guide. Lesions are targeted and the device base is adjusted accordingly to target the tumor. A sheath guide needle with an MR-compatible trocar is placed through the perineum into the target lesion using the grid. The trocar is removed and the laser fiber with an outer cooling cannula (with circulating saline and reflectors at the tip) is inserted through the sheath guide so that the target lesion is traversed. Additional scans are used to confirm the location of the applicator (96). After visualizing test pulses in MRI, and placing safety temperature point locations with adjacent thermal planes for monitoring, the laser is utilized to rapidly create an ablation zone with very discrete borders histologically (97). A cooling catheter may be used in the urethra. Temperatures of the rectal wall, periurethral zone, or perineural zones may be measured with direct thermocouple needles, or with MRI imaging, typically via proton resonance frequency-based methods. If these temperatures reach a pre-defined threshold for tissue damage, the laser apparatus will automatically shut off in order to protect the non-targeted tissue. The laser is activated for 90–120 s per ablation zone (typically 9 to 15 watts), and multiple overlapping ablations are done so that complete composite ablation of the planned treatment volume (incorporating the prostatic lesion) is ensured (96). Those patients who have prostatic lesions near the urethra, apex, sphincter, bladder neck, or nerves should be counseled on risk, and either safety maneuvers performed, or excluded from FLA in inexperienced hands, given the added risk for associated complications such as thermal injury, stricture, incontinence, erectile dysfunction, fistula, etc. During the ablation process, magnetic resonance temperature imaging (MRTI) is used real-time to monitor ablative progress using phase sensitive gradient-echo images which also allows for safe tissue coagulation of the lesion while helping to minimize collateral damage (97). A real-time temperature map is simultaneously displayed with a cumulative integral “death zone” of expected dead tissue, based upon the Arrhenius-like relationship of temperature and time versus bioeffects. A post-ablation scan is performed with intravenous Gadolinium and the ablation zones without enhancement indicate tissue necrosis (96). Post-treatment effects of FLA are demonstrated in Figures 3,4.
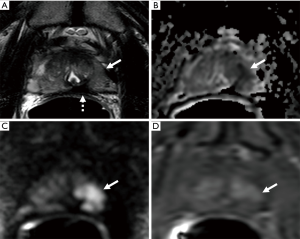
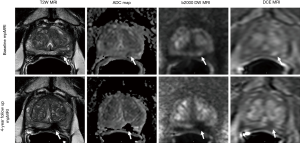
Whole gland prostate laser ablation studies from the 1980’s and 1990’s exist but outcomes research regarding prostate FLA are scarce (98). A phase I trial by Lindner and colleagues (99) in twelve patients with low-risk localized prostate cancer displayed that short-term morbidity was minimal with post-procedural, self-limiting perineal discomfort and mild hematuria as the most common adverse effects. Erectile function and LUTS were ultimately found to be unaffected. Post-procedural biopsy displayed that 50% of patients had no evidence of residual cancer and 67% were free of cancer at the ablation sites (99). A phase II trial by Eggener et al. (100) evaluated 27 men with stage T1c–T2a prostate cancer, Gleason 7 or less in 25% of biopsy specimens, PSA <15 ng/mL, and PSA density less than 0.15 ng/mL (3). A 3-month post-ablation MR guided biopsy of the ablation zones showed that 26/27 men had no evidence of prostate cancer within these zones. At 12 months, prostate cancer was identified in total ten patients, with cancer seen in the ablation zone in three (100). Hematuria was reported in 15%, perineal ecchymosis in 11%, and urinary retention in 8% (100).
Recent data display that prostate FLA is feasible, safe, and a viable option in eradicating prostate cancer in small focal lesions. However, oncologic outcomes both in the relative short-term and long-term need to be performed. Expected post-ablation PSA values and biopsy results need to be interpreted in the clinical context. Although there has been considerable patient-driven excitement for FLA, long-term results remain absent, as is the case for most local or image guided ablation therapies for focal prostate cancer.
Other therapies
Vascular-targeted photodynamic (VTP) therapy relies on a specific wavelength of light that is emitted from an optical fiber within the targeted prostate lesion. A water soluble vascular-targeting agent, WST11 (padeliporfin) or TOOKAD® (Steba Biotech, Luxemburg, Germany) is injected into the patient prior to the procedure which is then activated after coming in contact with the fibers emitting light at 753 nm (101). This causes the photosensitizing agent to generate reactive oxygen species leading to the destruction of tumor vasculature (102). In a trial by Taneja et al. (103), 30 men with low-risk (GS 6) and unilateral prostate cancer underwent mpMRI. Of those who underwent the calculated optimal dose of 4 mg/kg of WST11 at 200 J energy, 73.3% had a negative biopsy in the ablated lobe. Patients were followed for 1 year afterward with minimal sexual or urinary side effects (103). Another study by Azzouzi et al. (104) also showed similar rates of cancer control at short-term follow-up, 74% negative biopsies at 6 months, with little change in urinary or sexual function. Long-term studies comparing VTP to active surveillance are currently accruing (104).
Radiofrequency ablation (RFA) is a focal therapy in which a probe is introduced to within 0.5 mm of the desired location under TRUS guidance (105). Radiofrequency energy generates temperatures of approximately 100 °C to induce coagulation necrosis. While RFA has been used to successfully treat other types of cancer, experience with prostate cancer is limited. The technique was shown to be feasible via a transperineal approach under spinal or local anesthesia (106). These patients subsequently underwent prostatectomy and coagulation necrosis was seen in the expected regions with sparing of the periprostatic tissue (106,107). Multiple clinical trials are currently accruing LR and IR patients for evaluation of both cancer control and side effects of RFA, however there is currently a minimum of clinical data (105).
Convective water vapor is another ablative technique that is currently used to treat BPH by delivering thermal energy transurethrally to the central and peripheral zones of the prostate (108). After histological inspection, this energy has been shown to cause necrosis in the targeted lesions and a preliminary study with short (6 months) follow up has shown this to eliminate cancer both on confirmatory biopsy and mpMRI (109,110). Gold particles with ablation have been trialed in clinic as well, with the goal of selective uptake and heating of the tumor tissues, but peer reviewed results are pending.
Conclusions
The detection and imaging of prostate cancer has changed dramatically with advancements in mpMRI and fusion biopsy, and ablation technologies. While limitations do exist for mpMRI, this technology has led to improvements in the accuracy, adoption, and clinical utility of ambulatory prostate biopsy techniques and has allowed physicians to better characterize and better plan treatments. There has been a trend in more patients and physicians opting for active surveillance in the setting of low risk disease, with definitive therapy more reserved only for more aggressive prostate cancer. However, some patients who desire a “super-active surveillance” with ablation of an index lesion and interval MRI, PSA, and biopsy follow up. Others may be unwilling to undergo active surveillance. Some may desire to avoid the side effects of RT or RP, and some may need salvage therapy for radiation failures. When there are no widely agreed upon standard algorithms for some cohorts of patients with specific clinical situations, ages, PSAs, and certain histologies, specific patients may be ideal focal therapy candidates. While some data exist with oncological outcomes, additional long-term follow up is required to better define the roles for these therapies in routine clinical practice. Additional needs include more standardized monitoring after the procedure and clearer definitions of BCR. In addition to more follow up and data on focal therapy results, future research will explore additional detection modalities, such as PET imaging and multiparametric, ultra-high frequency, elastography, ultrasound thermometry, or contrast-enhanced ultrasound, as well as possible synergistic effects of adjuvant chemotherapeutics or immunotherapies (such as checkpoint inhibition) with cryoablation to enhance activity (111,112). MRI, fusion biopsy, and focal ablation with MRI or TRUS/MRI fusion guidance are changing the practice and management of patients with prostate cancer. Familiarity with recent developments and clinical trial results will better inform the field for the paradigm shifts that are already well underway. Although speculative, “super-active surveillance” has become a popular option for patients with specific low-grade prostate cancer pathology and distribution. Fusion guidance for focal ablation may also facilitate access for more patients to these emerging technologies at a lower overall public cost. Fusion and minimally invasive image guided therapies are rapidly defining roles for biopsy and ablation of certain prostate cancers.
Acknowledgements
This research was supported by the Intramural Research Program of the National Institutes of Health (NIH), National Cancer Institute, Center for Cancer Research, and the Center for Interventional Oncology.
Footnote
Conflicts of Interest: NIH and Philips Healthcare have a cooperative research and development agreement. NIH and Philips share intellectual property in the field. BJ Wood, PA Pinto, and B Turkbey receive royalties from Philips InVivo for licensed intellectual property in the field.
References
- SEER Cancer Stat Facts: Prostate Cancer. National Cancer Institute. Bethesda, MD. Available online: http://seer.cancer.gov/statfacts/html/prost.html
- Loeb S, Carter HB, Berndt SI, et al. Complications after prostate biopsy: data from SEER-Medicare. J Urol 2011;186:1830-4. [Crossref] [PubMed]
- Wilt TJ, Jones KM, Barry MJ, et al. Follow-up of Prostatectomy versus Observation for Early Prostate Cancer. N Engl J Med 2017;377:132-42. [Crossref] [PubMed]
- Ficarra V, Novara G, Rosen RC, et al. Systematic review and meta-analysis of studies reporting urinary continence recovery after robot-assisted radical prostatectomy. Eur Urol 2012;62:405-17. [Crossref] [PubMed]
- Ficarra V, Novara G, Ahlering TE, et al. Systematic review and meta-analysis of studies reporting potency rates after robot-assisted radical prostatectomy. Eur Urol 2012;62:418-30. [Crossref] [PubMed]
- Zaorsky NG, Shaikh T, Murphy CT, et al. Comparison of outcomes and toxicities among radiation therapy treatment options for prostate cancer. Cancer Treat Rev 2016;48:50-60. [Crossref] [PubMed]
- Siddiqui MM, Rais-Bahrami S, Turkbey B, et al. Comparison of MR/ultrasound fusion-guided biopsy with ultrasound-guided biopsy for the diagnosis of prostate cancer. JAMA 2015;313:390-7. [Crossref] [PubMed]
- San Francisco IF, DeWolf WC, Rosen S, et al. Extended prostate needle biopsy improves concordance of Gleason grading between prostate needle biopsy and radical prostatectomy. J Urol 2003;169:136-40. [Crossref] [PubMed]
- Quintana L, Ward A, Gerrin SJ, et al. Gleason Misclassification Rate Is Independent of Number of Biopsy Cores in Systematic Biopsy. Urology 2016;91:143-9. [Crossref] [PubMed]
- Bul M, Zhu X, Rannikko A, et al. Radical prostatectomy for low-risk prostate cancer following initial active surveillance: results from a prospective observational study. Eur Urol 2012;62:195-200. [Crossref] [PubMed]
- Taira AV, Merrick GS, Galbreath RW, et al. Performance of transperineal template-guided mapping biopsy in detecting prostate cancer in the initial and repeat biopsy setting. Prostate Cancer Prostatic Dis 2010;13:71-7. [Crossref] [PubMed]
- Mian BM, Lehr DJ, Moore CK, et al. Role of prostate biopsy schemes in accurate prediction of Gleason scores. Urology 2006;67:379-83. [Crossref] [PubMed]
- Rastinehad AR, Turkbey B, Salami SS, et al. Improving detection of clinically significant prostate cancer: magnetic resonance imaging/transrectal ultrasound fusion guided prostate biopsy. J Urol 2014;191:1749-54. [Crossref] [PubMed]
- Wysock JS, Rosenkrantz AB, Huang WC, et al. A prospective, blinded comparison of magnetic resonance (MR) imaging-ultrasound fusion and visual estimation in the performance of MR-targeted prostate biopsy: the PROFUS trial. Eur Urol 2014;66:343-51. [Crossref] [PubMed]
- Calio B, Sidana A, Sugano D, et al. Changes in prostate cancer detection rate of MRI-TRUS fusion vs systematic biopsy over time: evidence of a learning curve. Prostate Cancer Prostatic Dis 2017;20:436-41. [Crossref] [PubMed]
- Fütterer JJ, Briganti A, De Visschere P, et al. Can Clinically Significant Prostate Cancer Be Detected with Multiparametric Magnetic Resonance Imaging? A Systematic Review of the Literature. Eur Urol 2015;68:1045-53. [Crossref] [PubMed]
- Vargas HA, Hotker AM, Goldman DA, et al. Updated prostate imaging reporting and data system (PIRADS v2) recommendations for the detection of clinically significant prostate cancer using multiparametric MRI: critical evaluation using whole-mount pathology as standard of reference. Eur Radiol 2016;26:1606-12. [Crossref] [PubMed]
- Priester A, Natarajan S, Khoshnoodi P, et al. Magnetic Resonance Imaging Underestimation of Prostate Cancer Geometry: Use of Patient Specific Molds to Correlate Images with Whole Mount Pathology. J Urol 2017;197:320-6. [Crossref] [PubMed]
- D'Amico AV, Tempany CM, Cormack R, et al. Transperineal magnetic resonance image guided prostate biopsy. J Urol 2000;164:385-7. [Crossref] [PubMed]
- Sonn GA, Margolis DJ, Marks LS. Target detection: magnetic resonance imaging-ultrasound fusion-guided prostate biopsy. Urol Oncol 2014;32:903-11. [Crossref] [PubMed]
- Kwak JT, Hong CW, Pinto PA, et al. Is visual registration equivalent to semiautomated registration in prostate biopsy? Biomed Res Int 2015;2015:394742. [PubMed]
- Xu S, Kruecker J, Turkbey B, et al. Real-time MRI-TRUS fusion for guidance of targeted prostate biopsies. Comput Aided Surg 2008;13:255-64. [Crossref] [PubMed]
- Miyagawa T, Ishikawa S, Kimura T, et al. Real-time Virtual Sonography for navigation during targeted prostate biopsy using magnetic resonance imaging data. Int J Urol 2010;17:855-60. [Crossref] [PubMed]
- BK Fusion. Available online: https://bkultrasound.com/bkfusion-mr-fusion-biopsy/. Accessed August 23, 2017.
- Uronav. Available online: http://www.invivocorp.com/wp-content/uploads/2015/11/UroNav_Brochure.pdf. Accessed August 23, 2017.
- DK Technologies. Available online: http://dktech.de/. Accessed August 23, 2017.
- Focal Healthcare. Available online: http://www.focalhealthcare.com/fusion_biopsy_device/. Accessed August 23, 2017.
- Kongnyuy M, George AK, Rastinehad AR, et al. Magnetic Resonance Imaging-Ultrasound Fusion-Guided Prostate Biopsy: Review of Technology, Techniques, and Outcomes. Curr Urol Rep 2016;17:32. [Crossref] [PubMed]
- Biopsee. Available online: http://osl.uk.com/BiopSee. Accessed August 23, 2017.
- Trinity. Available online: http://koelis.com/en/products/Trinity/. Accessed August 23, 2017.
- Kongnyuy M, Siddiqui MM, George AK, et al. Multiparametric MRI/ultrasound fusion-guided biopsy decreases detection of indolent cancer in African-American men. Prostate Cancer Prostatic Dis 2017;20:348-51. [Crossref] [PubMed]
- ARTEMIS - 3D Ultrasound guided Prostate Biopsy Platform with MRI Fusion. Available online: http://www.innomedicus.com/index.asp?Language=EN&page=artemis. Accessed August 23, 2017.
- Frye TP, George AK, Kilchevsky A, et al. Magnetic Resonance Imaging-Transrectal Ultrasound Guided Fusion Biopsy to Detect Progression in Patients with Existing Lesions on Active Surveillance for Low and Intermediate Risk Prostate Cancer. J Urol 2017;197:640-6. [Crossref] [PubMed]
- Turkbey B, Mani H, Aras O, et al. Prostate cancer: can multiparametric MR imaging help identify patients who are candidates for active surveillance? Radiology 2013;268:144-52. [Crossref] [PubMed]
- Donaldson IA, Alonzi R, Barratt D, et al. Focal therapy: patients, interventions, and outcomes--a report from a consensus meeting. Eur Urol 2015;67:771-7. [Crossref] [PubMed]
- Eggener SE, Scardino PT, Carroll PR, et al. Focal therapy for localized prostate cancer: a critical appraisal of rationale and modalities. J Urol 2007;178:2260-7. [Crossref] [PubMed]
- National Comprehensive Cancer Network. Prostate Cancer (Version 2.2017). Available online: https://www.nccn.org/professionals/physician_gls/pdf/prostate.pdf. Accessed August 21, 2017.
- Truesdale MD, Cheetham PJ, Hruby GW, et al. An evaluation of patient selection criteria on predicting progression-free survival after primary focal unilateral nerve-sparing cryoablation for prostate cancer: recommendations for follow up. Cancer J 2010;16:544-9. [Crossref] [PubMed]
- Villers A, McNeal JE, Freiha FS, et al. Multiple cancers in the prostate. Morphologic features of clinically recognized versus incidental tumors. Cancer 1992;70:2313-8. [Crossref] [PubMed]
- Wise AM, Stamey TA, McNeal JE, et al. Morphologic and clinical significance of multifocal prostate cancers in radical prostatectomy specimens. Urology 2002;60:264-9. [Crossref] [PubMed]
- Mouraviev V, Mayes JM, Polascik TJ. Pathologic basis of focal therapy for early-stage prostate cancer. Nat Rev Urol 2009;6:205-15. [Crossref] [PubMed]
- Rukstalis DB, Goldknopf JL, Crowley EM, et al. Prostate cryoablation: a scientific rationale for future modifications. Urology 2002;60:19-25. [Crossref] [PubMed]
- Yin M, Bastacky S, Chandran U, et al. Prevalence of incidental prostate cancer in the general population: a study of healthy organ donors. J Urol 2008;179:892-5; discussion 895. [Crossref] [PubMed]
- Kabalin JN, McNeal JE, Price HM, et al. Unsuspected adenocarcinoma of the prostate in patients undergoing cystoprostatectomy for other causes: incidence, histology and morphometric observations. J Urol 1989;141:1091-4; discussion 1093-4. [Crossref] [PubMed]
- Noguchi M, Stamey TA, McNeal JE, et al. Prognostic factors for multifocal prostate cancer in radical prostatectomy specimens: lack of significance of secondary cancers. J Urol 2003;170:459-63. [Crossref] [PubMed]
- Sartor AO, Hricak H, Wheeler TM, et al. Evaluating localized prostate cancer and identifying candidates for focal therapy. Urology 2008;72:S12-24. [Crossref] [PubMed]
- Bott SR, Ahmed HU, Hindley RG, et al. The index lesion and focal therapy: an analysis of the pathological characteristics of prostate cancer. BJU Int 2010;106:1607-11. [Crossref] [PubMed]
- Liu W, Laitinen S, Khan S, et al. Copy number analysis indicates monoclonal origin of lethal metastatic prostate cancer. Nat Med 2009;15:559-65. [Crossref] [PubMed]
- Lucan VC, Lugnani F, Buttice S, et al. Cryotherapy for low risk prostate cancer, oncological and functional medium term outcomes: A three center prospective study. Arch Ital Urol Androl 2017;89:97-101. [Crossref] [PubMed]
- Onik G, Vaughan D, Lotenfoe R, et al. The "male lumpectomy": focal therapy for prostate cancer using cryoablation results in 48 patients with at least 2-year follow-up. Urol Oncol 2008;26:500-5. [Crossref] [PubMed]
- Ellis DS, Manny TB Jr, Rewcastle JC. Focal cryosurgery followed by penile rehabilitation as primary treatment for localized prostate cancer: initial results. Urology 2007;70:9-15. [Crossref] [PubMed]
- Ouzzane A, Betrouni N, Valerio M, et al. Focal therapy as primary treatment for localized prostate cancer: definition, needs and future. Future Oncol 2017;13:727-41. [Crossref] [PubMed]
- Onik G, Narayan P, Vaughan D, et al. Focal "nerve-sparing" cryosurgery for treatment of primary prostate cancer: a new approach to preserving potency. Urology 2002;60:109-14. [Crossref] [PubMed]
- Valerio M, Shah TT, Shah P, et al. Magnetic resonance imaging-transrectal ultrasound fusion focal cryotherapy of the prostate: A prospective development study. Urol Oncol 2017;35:150.e1-7. [Crossref] [PubMed]
- Tatsutani K, Rubinsky B, Onik G, et al. Effect of thermal variables on frozen human primary prostatic adenocarcinoma cells. Urology 1996;48:441-7. [Crossref] [PubMed]
- Bahn D, de Castro Abreu AL, Gill IS, et al. Focal cryotherapy for clinically unilateral, low-intermediate risk prostate cancer in 73 men with a median follow-up of 3.7 years. Eur Urol 2012;62:55-63. [Crossref] [PubMed]
- Barqawi AB, Stoimenova D, Krughoff K, et al. Targeted focal therapy for the management of organ confined prostate cancer. J Urol 2014;192:749-53. [Crossref] [PubMed]
- Gestaut MM, Cai W, Vyas S, et al. Low-Dose-Rate Brachytherapy Versus Cryotherapy in Low- and Intermediate-Risk Prostate Cancer. Int J Radiat Oncol Biol Phys 2017;98:101-7. [Crossref] [PubMed]
- Coleman JA, Scardino PT. Targeted prostate cancer ablation: energy options. Curr Opin Urol 2013;23:123-8. [Crossref] [PubMed]
- Sivaraman A, Barret E. Focal Therapy for Prostate Cancer: An "A la Carte" Approach. Eur Urol 2016;69:973-5. [Crossref] [PubMed]
- Durand M, Barret E, Galiano M, et al. Focal cryoablation: a treatment option for unilateral low-risk prostate cancer. BJU Int 2014;113:56-64. [Crossref] [PubMed]
- Hale Z, Miyake M, Palacios DA, et al. Focal cryosurgical ablation of the prostate: a single institute's perspective. BMC Urol 2013;13:2. [Crossref] [PubMed]
- Lynn JG, Putnam TJ. Histology of Cerebral Lesions Produced by Focused Ultrasound. Am J Pathol 1944;20:637-49. [PubMed]
- Chaussy C, Thuroff S, Rebillard X, et al. Technology insight: High-intensity focused ultrasound for urologic cancers. Nat Clin Pract Urol 2005;2:191-8. [Crossref] [PubMed]
- Madersbacher S, Kratzik C, Szabo N, et al. Tissue ablation in benign prostatic hyperplasia with high-intensity focused ultrasound. Eur Urol 1993;23 Suppl 1:39-43. [Crossref] [PubMed]
- Schulman AA, Tay KJ, Robertson CN, et al. High-intensity focused ultrasound for focal therapy: reality or pitfall? Curr Opin Urol 2017;27:138-48. [Crossref] [PubMed]
- Barkin J. High intensity focused ultrasound (HIFU). Can J Urol 2011;18:5634-43. [PubMed]
- Blana A, Brown SC, Chaussy C, et al. High-intensity focused ultrasound for prostate cancer: comparative definitions of biochemical failure. BJU Int 2009;104:1058-62. [Crossref] [PubMed]
- Ganzer R, Fritsche HM, Brandtner A, et al. Fourteen-year oncological and functional outcomes of high-intensity focused ultrasound in localized prostate cancer. BJU Int 2013;112:322-9. [Crossref] [PubMed]
- Pinthus JH, Farrokhyar F, Hassouna MM, et al. Single-session primary high-intensity focused ultrasonography treatment for localized prostate cancer: biochemical outcomes using third generation-based technology. BJU Int 2012;110:1142-8. [Crossref] [PubMed]
- Crouzet S, Chapelon JY, Rouviere O, et al. Whole-gland ablation of localized prostate cancer with high-intensity focused ultrasound: oncologic outcomes and morbidity in 1002 patients. Eur Urol 2014;65:907-14. [Crossref] [PubMed]
- Rischmann P, Gelet A, Riche B, et al. Focal High Intensity Focused Ultrasound of Unilateral Localized Prostate Cancer: A Prospective Multicentric Hemiablation Study of 111 Patients. Eur Urol 2017;71:267-73. [Crossref] [PubMed]
- van Velthoven R, Aoun F, Marcelis Q, et al. A prospective clinical trial of HIFU hemiablation for clinically localized prostate cancer. Prostate Cancer Prostatic Dis 2016;19:79-83. [Crossref] [PubMed]
- Feijoo ER, Sivaraman A, Barret E, et al. Focal High-intensity Focused Ultrasound Targeted Hemiablation for Unilateral Prostate Cancer: A Prospective Evaluation of Oncologic and Functional Outcomes. Eur Urol 2016;69:214-20. [Crossref] [PubMed]
- El Fegoun AB, Barret E, Prapotnich D, et al. Focal therapy with high-intensity focused ultrasound for prostate cancer in the elderly. A feasibility study with 10 years follow-up. Int Braz J Urol 2011;37:213-9; discussion 220-2. [Crossref] [PubMed]
- Ahmed HU, Freeman A, Kirkham A, et al. Focal therapy for localized prostate cancer: a phase I/II trial. J Urol 2011;185:1246-54. [Crossref] [PubMed]
- Murray KS, Ehdaie B, Musser J, et al. Pilot Study to Assess Safety and Clinical Outcomes of Irreversible Electroporation for Partial Gland Ablation in Men with Prostate Cancer. J Urol 2016;196:883-90. [Crossref] [PubMed]
- Scheltema MJ, Postema AW, de Bruin DM, et al. Irreversible electroporation for the treatment of localized prostate cancer: a summary of imaging findings and treatment feedback. Diagn Interv Radiol 2017;23:365-70. [Crossref] [PubMed]
- Valerio M, Dickinson L, Ali A, et al. Nanoknife Electroporation Ablation Trial: A Prospective Development Study Investigating Focal Irreversible Electroporation for Localized Prostate Cancer. J Urol 2017;197:647-54. [Crossref] [PubMed]
- Ahmed HU, Hindley RG, Dickinson L, et al. Focal therapy for localised unifocal and multifocal prostate cancer: a prospective development study. Lancet Oncol 2012;13:622-32. [Crossref] [PubMed]
- Ahmed HU, Dickinson L, Charman S, et al. Focal Ablation Targeted to the Index Lesion in Multifocal Localised Prostate Cancer: a Prospective Development Study. Eur Urol 2015;68:927-36. [Crossref] [PubMed]
- Ahmed HU, Zacharakis E, Dudderidge T, et al. High-intensity-focused ultrasound in the treatment of primary prostate cancer: the first UK series. Br J Cancer 2009;101:19-26. [Crossref] [PubMed]
- Dickinson L, Arya M, Afzal N, et al. Medium-term Outcomes after Whole-gland High-intensity Focused Ultrasound for the Treatment of Nonmetastatic Prostate Cancer from a Multicentre Registry Cohort. Eur Urol 2016;70:668-74. [Crossref] [PubMed]
- Ahmed HU, Cathcart P, McCartan N, et al. Focal salvage therapy for localized prostate cancer recurrence after external beam radiotherapy: a pilot study. Cancer 2012;118:4148-55. [Crossref] [PubMed]
- Baco E, Gelet A, Crouzet S, et al. Hemi salvage high-intensity focused ultrasound (HIFU) in unilateral radiorecurrent prostate cancer: a prospective two-centre study. BJU Int 2014;114:532-40. [Crossref] [PubMed]
- van den Bos W, Scheltema MJ, Siriwardana AR, et al. Focal irreversible electroporation as primary treatment for localized prostate cancer. BJU Int 2017. [Epub ahead of print]. [Crossref] [PubMed]
- Stanevsky Y, Tsivian A, Tsivian M. Castration-resistant prostate cancer: a strategy to enhance response to androgen deprivation. Asian J Androl 2013;15:709-10. [Crossref] [PubMed]
- Turjanski P, Olaiz N, Maglietti F, et al. The role of pH fronts in reversible electroporation. PLoS One 2011;6:e17303. [Crossref] [PubMed]
- Valerio M, Ahmed HU, Emberton M. Focal Therapy of Prostate Cancer Using Irreversible Electroporation. Tech Vasc Interv Radiol 2015;18:147-52. [Crossref] [PubMed]
- Scheltema MJ, van den Bos W, Siriwardana AR, et al. Feasibility and safety of focal irreversible electroporation as salvage treatment for localized radio-recurrent prostate cancer. BJU Int 2017;120 Suppl 3:51-8. [Crossref] [PubMed]
- Baur ADJ, Collettini F, Enders J, et al. MRI-TRUS fusion for electrode positioning during irreversible electroporation for treatment of prostate cancer. Diagn Interv Radiol 2017;23:321-5. [Crossref] [PubMed]
- van den Bos W, de Bruin DM, van Randen A, et al. MRI and contrast-enhanced ultrasound imaging for evaluation of focal irreversible electroporation treatment: results from a phase I-II study in patients undergoing IRE followed by radical prostatectomy. Eur Radiol 2016;26:2252-60. [Crossref] [PubMed]
- van den Bos W, Jurhill RR, de Bruin DM, et al. Histopathological Outcomes after Irreversible Electroporation for Prostate Cancer: Results of an Ablate and Resect Study. J Urol 2016;196:552-9. [Crossref] [PubMed]
- Lindner U, Lawrentschuk N, Trachtenberg J. Focal laser ablation for localized prostate cancer. J Endourol 2010;24:791-7. [Crossref] [PubMed]
- Huang GT, Wang TH, Sheu JC, et al. Low-power laserthermia for the treatment of small hepatocellular carcinoma. Eur J Cancer 1991;27:1622-7. [Crossref] [PubMed]
- Lee T, Mendhiratta N, Sperling D, et al. Focal laser ablation for localized prostate cancer: principles, clinical trials, and our initial experience. Rev Urol 2014;16:55-66. [PubMed]
- Stafford RJ, Shetty A, Elliott AM, et al. Magnetic resonance guided, focal laser induced interstitial thermal therapy in a canine prostate model. J Urol 2010;184:1514-20. [Crossref] [PubMed]
- Lindner U, Trachtenberg J, Lawrentschuk N. Focal therapy in prostate cancer: modalities, findings and future considerations. Nat Rev Urol 2010;7:562-71. [Crossref] [PubMed]
- Lindner U, Weersink RA, Haider MA, et al. Image guided photothermal focal therapy for localized prostate cancer: phase I trial. J Urol 2009;182:1371-7. [Crossref] [PubMed]
- Eggener SE, Yousuf A, Watson S, et al. Phase II Evaluation of Magnetic Resonance Imaging Guided Focal Laser Ablation of Prostate Cancer. J Urol 2016;196:1670-5. [Crossref] [PubMed]
- Betrouni N, Lopes R, Puech P, et al. A model to estimate the outcome of prostate cancer photodynamic therapy with TOOKAD Soluble WST11. Phys Med Biol 2011;56:4771-83. [Crossref] [PubMed]
- Trachtenberg J, Weersink RA, Davidson SR, et al. Vascular-targeted photodynamic therapy (padoporfin, WST09) for recurrent prostate cancer after failure of external beam radiotherapy: a study of escalating light doses. BJU Int 2008;102:556-62. [Crossref] [PubMed]
- Taneja SS, Bennett J, Coleman J, et al. Final Results of a Phase I/II Multicenter Trial of WST11 Vascular Targeted Photodynamic Therapy for Hemi-Ablation of the Prostate in Men with Unilateral Low Risk Prostate Cancer Performed in the United States. J Urol 2016;196:1096-104. [Crossref] [PubMed]
- Azzouzi AR, Barret E, Bennet J, et al. TOOKAD(R) Soluble focal therapy: pooled analysis of three phase II studies assessing the minimally invasive ablation of localized prostate cancer. World J Urol 2015;33:945-53. [Crossref] [PubMed]
- Valerio M, Cerantola Y, Eggener SE, et al. New and Established Technology in Focal Ablation of the Prostate: A Systematic Review. Eur Urol 2017;71:17-34. [Crossref] [PubMed]
- Zlotta AR, Djavan B, Matos C, et al. Percutaneous transperineal radiofrequency ablation of prostate tumour: safety, feasibility and pathological effects on human prostate cancer. Br J Urol 1998;81:265-75. [Crossref] [PubMed]
- Djavan B, Susani M, Shariat S, et al. Transperineal radiofrequency interstitial tumor ablation (RITA) of the prostate. Tech Urol 1998;4:103-9. [PubMed]
- Roehrborn CG, Gange SN, Gittelman MC, et al. Convective Thermal Therapy: Durable 2-Year Results of Randomized Controlled and Prospective Crossover Studies for Treatment of Lower Urinary Tract Symptoms Due to Benign Prostatic Hyperplasia. J Urol 2017;197:1507-16. [Crossref] [PubMed]
- Dixon CM, Rijo Cedano E, Mynderse LA, et al. Transurethral convective water vapor as a treatment for lower urinary tract symptomatology due to benign prostatic hyperplasia using the Rezum((R)) system: evaluation of acute ablative capabilities in the human prostate. Res Rep Urol 2015;7:13-8. [Crossref] [PubMed]
- Dixon C, Lay R, Cabanas C, et al. Development of Convective Water Vapor Energy for Treating Localized Prostate Cancer: First-in-Man Early Clinical Experiences. American Urological Association Boston, MA, 2017.
- Baust JG, Bischof JC, Jiang-Hughes S, et al. Re-purposing cryoablation: a combinatorial 'therapy' for the destruction of tissue. Prostate Cancer Prostatic Dis 2015;18:87-95. [Crossref] [PubMed]
- Tay KJ, Schulman AA, Sze C, et al. New advances in focal therapy for early stage prostate cancer. Expert Rev Anticancer Ther 2017;17:737-43. [Crossref] [PubMed]