Opportunities and challenges of intermittent and continuous intraoperative neural monitoring in thyroid surgery
Introduction
Nerve monitoring is a complex process that encompasses three interdependent steps of RLN evaluation: preoperative, intraoperative, and postoperative assessment of RLN function. Intraoperative neural monitoring (IONM) hinges on the accurate measurement and correct interpretation of two electrophysiological key parameters: nerve amplitude and nerve latency. Both are elicited through stimulation of the vagus nerve (VN) and respectively the recurrent laryngeal nerve (RLN) and the external branch of the superior laryngeal nerve (EBSLN). Beyond that electrophysiological level, measurements of voice, breathing and swallowing can have a major impact on a patient’s quality of life, coming at the top of the pyramid of RLN integrity (Figure 1). IONM is not devoid of methodological limitations, which need to be accounted for. This review summarizes the current key achievements of IONM; outlines opportunities for improvement regarding clinical implementation; and suggests areas of future research in this rapidly evolving field.
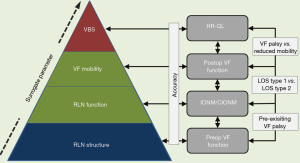
The IONM formula standard: room for improvement
Although IONM has been widely used, the clinical application of IONM and the interpretation of electrophysiology signals can vary tremendously across clinical settings. To remedy this deficiency, active efforts are being made to launch a series of training courses emphasizing the need to use intermittent IONM and continuous IONM (CIONM) in a standard fashion (1).
For standardization of IONM, the International Neural Monitoring Study Group has proposed the L1–V1–R1–R2–V2–L2 formula that entails the following sequence of steps (2):
L1: evaluation of preoperative vocal fold (VF) function on laryngoscopy;
V1: verification of VN functional integrity before dissection;
R1: verification of RLN functional integrity before dissection;
R2: verification of RLN functional integrity after dissection;
V2: verification of VN functional integrity after dissection;
L2: evaluation of postoperative VF function on laryngoscopy.
The L1–V1–R1–R2–V2–L2 formula is applicable to both intermittent IONM and CIONM. By definition, CIONM cannot be performed unless the vagal nerve is stimulated, rendering V2 important for intraoperative prediction of postoperative VF palsy (3,4).
Standardization of intermittent IONM and CIONM is important to ensure reproducible and reliable results (5). Preoperative and postoperative laryngeal examination to ascertain baseline VF function clinically before the operation is critical because the action potential of the stimulated nerve is no more than a surrogate parameter suggestive of, but not definitively proving, normal VF function. There is general consensus that a reliable functional nerve assessment is unfeasible without having clinical confirmation that VF mobility has been intact from the outset (6). Among 285 patients who had VF palsy from the very beginning, 36 patients (13%) had a completely normal voice, whereas 41 patients (14.3%) with some degree of hoarseness revealed abnormal IONM signals with preserved nerve conductivity (Figure 2A). This is why preoperative laryngoscopy is an integral element of IONM, even in the presence of a normal voice (7).
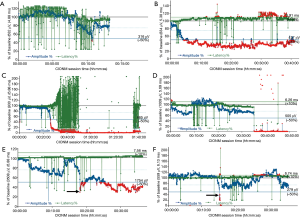
Clinical practice has been a different story, though. A Swedish study of 3,277 patients published in 2016 revealed that laryngoscopy was performed in 44.3% before and only 29.6% after thyroidectomy under IONM (8). Patients who underwent postoperative laryngoscopy as a matter of routine had fewer permanent postoperative VF palsies than patients without routine laryngoscopy (1.6% vs. 5.9%). As a matter of fact, intraoperative stimulation of the VN affords reliable distinction between RLN lesions and technical artefacts. Yet a 2012 survey on usage patterns with nerve monitoring revealed that vagal stimulation was routinely carried out in Germany in 49.3% of patients before and 73.8% of patients after thyroidectomy only (9). Higher rates of routine vagal stimulation before and after resection were associated with an institution’s frequent use of RLN monitoring and higher surgical caseload.
Challenges of implementation and technological pitfalls of IONM
In many parts of the world, the uptake of IONM for thyroid surgery is rising, in particular in Europe (Germany, Italy, Denmark, Poland, France, Spain, UK), the US and Asia, specifically China (10). Some 53% of general surgeons and up to 65% of otolaryngologists in the United States reportedly used intermittent IONM for some or all thyroid operations (11,12), whereas German surgical departments indicated that more than 92% of their staff surgeons routinely used intermittent IONM during thyroidectomy (9). There are no corresponding data for CIONM, which may have been used in 2015 on almost 10% of all thyroid operations in Germany based on personal information (unpublished data). In the United States, the rationale for using IONM is split along professional lines: general surgeons prefer IONM to locate the RLN, whereas otolaryngologists use IONM more often for monitoring the RLN during thyroid resection and for documentation purposes to guard against malpractice claims (1).
In 2011, the International Neural Monitoring Study Group laid down standards for the set-up of IONM equipment; provided definitions for incomplete and complete loss of the electromyographical signal (LOS); and proposed an intraoperative trouble-shooting algorithm (2). Equipment malfunction and endotracheal tube dislocation or rotation (Figure 2B), and the choice of an inappropriately sized tube can alter the electrophysiological signal and cause misinterpretation, potentially putting the RLN at jeopardy (13-16). In CIONM (as opposed to intermittent IONM), the baseline amplitude is calibrated such that the highest possible amplitude is obtained by maximizing the contact of the recording electrodes on the orotracheal tube with the glottis wall. This is why mean baseline amplitude are much higher for CIONM: 945 µV based on 1,535 patients with 2,635 nerves at risk (17), as compared to the reference for intermittent IONM of 460 µV (18). Intraoperative nerve amplitudes are then gauged against that maximum baseline amplitude.
For meaningful interpretation of signals, baseline amplitudes need to be at least ≥500 µV after supra-threshold stimulation (1 mA) of the ipsilateral VN (19,20):
- Electrophysiological artefacts, featuring a reciprocal relationship between amplitude and latency, may be misconstrued when the baseline amplitude is <500 µV;
- Receiver operating characteristic analysis for absolute loss of signal yielded an amplitude threshold of 259 µV to predict early postoperative VF palsy (17).
With the advent of intermittent IONM, surgeons initially were on a steep learning curve before becoming more familiar with the technology (13,14). This learning curve arguably may be steeper for CIONM, because the operating surgeon needs to constantly monitoring the electrophysiological response signals (21). Electrophysiological artefacts in amplitude, latency and waveform have little, if any, bearing on intermittent IONM (absent the use of the handheld stimulation probe), except for so-called ‘low amplitude waveforms’. Low amplitude waveforms from bystander (non-target) muscles can be explained by the concept of far-field artefactual waveforms and do not reflect a true electrophysiological response from these muscles (22). For CIONM, the situation is completely different because artefacts pretending to show amplitude decreases in conjunction with latency increases can mimic so-called ‘combined events’ that herald impending nerve injury (Figure 2B) (15,20).
System malfunction can compromise the utility of CIONM. In one study, at least one system alert was triggered for every other high-risk nerve dissected, even though the baseline amplitude was as high as >300 µV (23). Among 138 events reported, 91 events (66%) were classified as technical artefacts due to minor surgical maneuvers. Also noted were nerve lesions secondary to the vagus electrode, including one reversible nerve lesion situated above the level of the vagal electrode. Two serious adverse events, hemodynamic instability and reversible vagal neuropraxia, during CIONM were described in a small series of 9 patients with 12 nerves at risk (24). Intermittent VN stimulation also has been implicated in 2 anecdotal reports as the cause of asystoly leading to cardiac arrest (25).
These limited reports are at variance with the experience of a large national referral center based on 1,800 patients with 3,049 nerves at risk, in whom no clinically relevant changes in heart rate or blood pressure, cardiac arrhythmia, or other hemodynamically important events were encountered (26). On intensive monitoring of a high-risk subgroup of 6 patients with 12 nerves at risk for pre-existent second- or third-degree atrioventricular block, systolic and diastolic blood pressures, heart rate, and peripheral arterial oxygen saturation remained within normal limits before, during, and after CIONM (26). Although CIONM augments vagal activity subclinically, this does not set off any counterregulation by the sympathetic nervous system (27). Experimental and clinical studies that investigated the issue more systematically did not uncover meaningful cardiac, pulmonary, or gastric vagal adverse effects (20,28-32).
In recent years, endoscopic and robotic surgery has been on the rise, notably in Asia (33). A recent review of the literature found that IONM was employed in no more than 9 (5%) of 160 studies dealing with endoscopic and robotic surgery (34). Only 3 (30%) of these 9 studies also entailed stimulation of the VN, and only 2 studies also stimulation of the EBSLN. Unlike intermittent IONM that requires stimulation from a remote access, CIONM can be integrated more easily into single-incision transaxillary robotic thyroidectomy (33).
Challenges of preventing RLN palsy under IONM
The independent contribution of IONM to the prevention of RLN palsy is one of the most hotly debated themes in the field of thyroid surgery (35-37). Meta-analyses of studies, many of which were limited by poor study design and the sole use of intermittent nerve stimulation, were unable to demonstrate superiority of IONM over mere anatomic RLN dissection (38-41). Recent systematic reviews pointed towards a benefit of IONM in decreasing RLN palsy rates, but failed to reach statistical significance for persistent RLN palsy (42,43). A recent reanalysis of the Scandinavian Quality Register for Thyroid, Parathyroid and Adrenal Surgery found that the use of IONM was not independently associated with a lower risk of early vocal cord palsy (odds ratio 0.67; 95% confidence interval, 0.44–1.01), but independently decreased the risk of permanent vocal cord palsy (odds ratio 0.43; 95% confidence interval, 0.19–0.93) (8). There is still a paucity of prospective randomized clinical trials. Only one randomized prospective study found a statistically significant benefit of IONM for prevention of temporary RLN palsy (44).
Surgeons need to be cognizant of the methodological limitations of intermittent IONM in both time and space:
- Limited in time because the period between the stimulation cycles goes unsupervised, at which time the RLN continues to be at jeopardy;
- Limited in space because RLN function is monitored only between the levels of the stimulation and recording electrodes, missing proximal RLN lesions.
Despite these methodological limitations, intermittent IONM is able to reliably predict postoperative VF palsy after the RLN has been injured.
CIONM may be a more prudent approach than intermittent IONM (42). Indeed, an observational study of 1,526 patients confirmed that operation with CIONM resulted in fewer permanent VF palsies compared with intermittent IONM (0 vs. 0.4%; P=0.019) after thyroid surgery in patients with benign disease (4). CIONM helps detect adverse EMG changes early on and prevent severe RLN injuries that cause LOS by permitting the surgeon to release distressed nerves (20,45,46).
RLN injury comes in two varieties, which differ in many ways (47):
- Segmental LOS type 1: represents rapid onset, severe RLN injury, caused by traction (68%), electrocoagulation around the nerve (16%) or pinching (13%) and associated with less frequent and slower recovery of VF dysfunction;
- Global LOS type 2: signals more gradual and milder RLN injury, caused by traction (92%) and associated with more frequent and faster recovery of VF dysfunction.
CIONM is well suited to document the gradual development of global LOS type 2 under indirect traction on the nerve. So-called ‘combined events’, the combination of a >50% decrease in amplitude coupled with a >110% increase in latency relative to baseline, precede this type of RLN injury, which commonly resolves on prompt reversal of the causative surgical maneuver (Figure 2C). In contrast, segmental LOS type 1 manifests abruptly upon stretching, coagulation around, and pinching the nerve, causing instant damage that often translates into complete early VF palsy (Figure 2D). In segmental LOS type 1 injury, latency basically remains unchanged whereas the corresponding amplitude plummets owing to the acute nature of the injury. Segmental LOS type 1 sets in more abruptly than global LOS type 2 (156.0 vs. 2.0 s; P<0.001), reflecting the greater severity of RLN injury (17). By implication, there is a window of opportunity within which RLN injury can be averted. With growing experience, even abrupt declines in amplitude, typically caused by electrocoagulation, become increasingly reversible (personal experience, Figure 2E). The window of opportunity closes when the premonitory ‘combined events’ degrade to definite LOS.
Challenges of predicting RLN palsy
The clinical utility of IONM can be expressed in terms of its negative (NPV) and positive predictive values (PPV). Owing to the rarity of RLN palsy, NPV and specificity for intermittent IONM are high at 97.4% and 97.8%, whereas PPV and sensitivity are lower at 63.4% and 66.8% (42). Strict adherence to IONM standards, avoiding technical pitfalls, can improve NPV and PPV to 99.8% and 78.4%, respectively (4). Increases in the sensitivity of intermittent IONM over time have been paralleled by a steady decrease of early postoperative VF palsy rates from >8.7% in 2004 to ≤2.4% currently. The low rates of false-negative results (Figure 2F) attest to the high level of safety at expert referral centers for endocrine surgery. CIONM, featuring a NPV of 99.8%, a PPV of 88.2% and a sensitivity of 90.9%, nevertheless may produce false-positive results in the absence of RLN palsy, which may delay completion thyroidectomy of the other thyroid lobe (Figure 2C) (48).
Another point to be considered is intraoperative recovery of the electrophysiological signal after loss of signal during a 20-min waiting period. This strategy of expectant observation carries a ≥20% residual risk of transient VF palsy despite intraoperative normalization of the EMG signal (17). Intraoperative recovery of the electrophysiological signal ≥50% of baseline always signifies normal postoperative VF function, whereas signal recovery <50% of baseline indicates early VF palsy in all patients with segmental type 1 and in two thirds of patients with global type 2 injuries. Persistent LOS heralded VF palsy in all patients with segmental type 1 injury and three-quarters of patients with global type 2 injury. These findings suggest that an injured nerve be given a minimum wait of 20 minutes after LOS has set in to recover ≥50% of its baseline amplitude before moving on with completion thyroidectomy on the unaffected side (48). Indeed, as many as 85% of segmental RLN injuries type 1 and 94% of global RLN injuries type 2 recovered completely within half a year (47).
Challenges of IONM of the EBSLN
In 2013, the International Neural Monitoring Study Group published a guideline on IONM of the EBSLN (49). Compared to RLN injury, the clinical sequelae of EBSLN injury, being clinically less apparent, are frequently underestimated. The resultant dysphonia can have a profound impact on singers who professionally may be dependent on their voice. The EBSLN is as susceptible to injury as is the RLN through the same mechanisms of injury. IONM may help prevent EBLSM injury by identifying the nerve intraoperatively, which is successful 80% of the time (50). Lowering the positive threshold level to 50 or 100 µV may improve EBSLN detection. Because the EBSLN innervates the anterior one-third of the glottis, making closer contact between the nerve and standard endotracheal electrode can be difficult. Newer endotracheal tube designs provide for larger circumferential electrode surface areas to facilitate detection of anterior glottis depolarization (51-53). The role of VN stimulation for IONM of the EBSLN, which is pivotal for IONM of the RLN, remains to be defined.
Cost-effectiveness of IONM: an economic challenge
A recent cost-utility analysis of RLN monitoring used a decision-tree model of total thyroidectomy to analyze the cost-effectiveness of three clinical scenarios from a societal perspective (54): (I) routine IONM; versus (II) selective IONM for high-risk operations including reoperations, substernal or toxic goiters, and cases with known cancer; versus (III) visual identification of the RLN only. In this model, visual identification of the RLN was more cost-effective than any use of IONM. If a clinician can, with the use of IONM, decrease the rate of RLN injury by 50.4% or more compared with visual identification, the selective use of IONM in high-risk cases was most cost-effective. Equipment and consumables for IONM, especially CIONM, are expensive and very country-specific. The IONM technique can hardly be cost-effective when only the direct costs of RLN injuries are considered (55). To be cost-effective, all indirect costs of RLN injuries, including speech therapy, repeat laryngeal examinations, VF surgery, and legal claims, must be factored into the cost-effectiveness equation. A most recent analysis validated IONM utilization as a cost-effective measure in patients undergoing bilateral thyroidectomy (56). Inpatient costs for all forms of thyroidectomy, regardless of the equipment used, continue to be underfunded. The basic Diagnosis-Related Group (DRG) for thyroidectomy need to properly reflect the actual costs incurred by the service providers if the situation were to change. Greater demand may also set greater economic incentives for more competition in the medical device arena. Basic Diagnosis-Related Group (DRG) reimbursement is more geared at containing costs than encouraging the use of novel technology (10).
Future perspectives
Animal models have been instrumental in understanding neural electrophysiology and the related IONM technology, clarifying the effects of direct and indirect nerve injury on nerve electrophysiology and the vocalis muscle (31). Further research should be targeted at further clarifying causation, histopathological nerve damage, and the clinical consequences of segmental nerve injury type 1 and global nerve injury type 2 (17,47). On a cautionary note, animal studies may not be fully generalizable to humans, leaving room for interpretation (31). Joint electrophysiological, histopathological, and clinical analyses of RLN injury induced in a porcine model under CIONM led to a classification system of RLN injury according to severity that now requires prospective validation in humans (57). Importantly, animal models cannot mimic human thyroid disease and differ greatly from humans in terms of laryngeal anatomy, formation of voice, as well as breathing and swallowing. In a sense, IONM is like a new language that needs to be learned (20). Particular attention should be placed on the occasional disconnect between RLN function and VF mobility, which is still incompletely understood. Health-related quality of life hinges on the preservation of voice, breathing, and swallowing. For voice, breathing and swallowing, VF motility is the best single surrogate parameter, which is best predicted intraoperatively by the electrophysiological IONM signal (Figure 1) (20).
Conclusions
The number of thyroid operations continues to rise in the western hemisphere, bringing prevention of RLN palsy to the fore. Overall, the incidence of RLN palsy is fairly low but continues to prompt litigation for malpractice. In an effort to diminish transient, and more importantly permanent, RLN palsy rates, intraoperative neuromonitoring (IONM) has been advocated as a risk minimization tool. Recent meta-analyses of studies, many of which were limited by poor study design and the sole use of intermittent nerve stimulation, were unable to demonstrate superiority of IONM over mere anatomic RLN dissection. This is where CIONM comes into play: This technology enables the surgeon to (I) spot impending nerve injury as it unfolds; (II) release distressed nerves by reversing causative surgical maneuvers; and (III) verify functional nerve recovery after intraoperative loss of the electromyographic signal. Despite this superiority, CIONM is not devoid of methodological limitations, which need to be accounted for. This review summarizes the current key achievements of IONM; outlines opportunities for improvement regarding clinical implementation; and suggests areas of future research in this rapidly evolving field.
Acknowledgements
None.
Footnote
Conflicts of Interest: H Dralle was remunerated by Medtronic and Inomed for giving lectures on intraoperative nerve monitoring.
References
- Ho Y, Carr MM, Goldenberg D. Trends in intraoperative neural monitoring for thyroid and parathyroid surgery amongst otolaryngologists and general surgeons. Eur Arch Otorhinolaryngol 2013;270:2525-30. [Crossref] [PubMed]
- Randolph GW, Dralle H. International Neuromonitoring Stuy Group. Electrophysiologic recurrent laryngeal nerve monitoring during thyroid and parathyroid surgery: international standards guideline statement. Laryngoscope 2011;121 Suppl 1:S1-16. [Crossref] [PubMed]
- Hermann M, Hellebart C, Freissmuth M. Neuromonitoring in thyroid surgery: prospective evaluation of intraoperative electrophysiological responses for the prediction of recurrent laryngeal nerve injury. Ann Surg 2004;240:9-17. [Crossref] [PubMed]
- Schneider R, Sekulla C, Machens A, et al. Postoperative vocal fold palsy in patients undergoing thyroid surgery with continuous or intermittent nerve monitoring. Br J Surg 2015;102:1380-7. [Crossref] [PubMed]
- Chiang FY, Lee KW, Chen HC, et al. Standardization of intraoperative neuromonitoring of recurrent laryngeal nerve in thyroid operation. World J Surg 2010;34:223-9. [Crossref] [PubMed]
- Lorenz K, Abuazab M, Sekulla C, et al. Results of intraoperative neuromonitoring in thyroid surgery and preoperative vocal cord paralysis. World J Surg 2014;38:582-91. [Crossref] [PubMed]
- Sinclair CF, Bumpous JM, Haugen BR, et al. Laryngeal examination in thyroid and parathyroid surgery: An American Head and Neck Society consensus statement: AHNS Consensus Statement. Head Neck 2016;38:811-9. [Crossref] [PubMed]
- Bergenfelz A, Salem AF, Jacobsson H, et al. Risk of recurrent laryngeal nerve palsy in patients undergoing thyroidectomy with and without intraoperative nerve monitoring. Br J Surg 2016;103:1828-38. [Crossref] [PubMed]
- Dralle H, Sekulla C, Lorenz K, et al. Loss of the nerve monitoring signal during bilateral thyroid surgery. Br J Surg 2012;99:1089-95. [Crossref] [PubMed]
- Dionigi G, Lombardi D, Lombardi CP, et al. Intraoperative neuromonitoring in thyroid surgery: a point prevalence survey on utilization, management, and documentation in Italy. Updates Surg 2014;66:269-76. [Crossref] [PubMed]
- Singer MC, Rosenfeld RM, Sundaram K. Laryngeal nerve monitoring: current utilization among head and neck surgeons. Otolaryngol Head Neck Surg 2012;146:895-9. [Crossref] [PubMed]
- Sturgeon C, Sturgeon T, Angelos P. Neuromonitoring in thyroid surgery: attitudes, usage patterns, and predictors of use among endocrine surgeons. World J Surg 2009;33:417-25. [Crossref] [PubMed]
- Dionigi G, Bacuzzi A, Boni L, et al. What is the learning curve for intraoperative neuromonitoring in thyroid surgery? Int J Surg 2008;6:S7-12. [Crossref] [PubMed]
- Dionigi G, Donatini G, Boni L, et al. Continuous monitoring of the recurrent laryngeal nerve in thyroid surgery: a critical appraisal. Int J Surg 2013;11:S44-6. [Crossref] [PubMed]
- Schneider R, Lorenz K, Machens A, et al. Continuous intraoperative neruomonitoring (CIONM) of the recurrent laryngeal nerve. In: Randolph GW, editor. The Recurrent and Superior Laryngeal Nerves, Springer International Switzerland 2016:169-83.
- Wu CW, Wang MH, Chen CC, et al. Loss of signal in recurrent nerve neuromonitoring: causes and management. Gland Surg 2015;4:19-26. [PubMed]
- Schneider R, Sekulla C, Machens A, et al. Dynamics of loss and recovery of the nerve monitoring signal during thyroidectomy predict early postoperative vocal fold function. Head Neck 2016;38:E1144-51. [Crossref] [PubMed]
- Lorenz K, Sekulla C, Schelle J, et al. What are normal quantitative parameters of intraoperative neuromonitoring (IONM) in thyroid surgery? Langenbecks Arch Surg 2010;395:901-9. [Crossref] [PubMed]
- Schneider R, Randolph GW, Sekulla C, et al. Continuous intraoperative vagus nerve stimulation for identification of imminent recurrent laryngeal nerve injury. Head Neck 2013;35:1591-8. [Crossref] [PubMed]
- Schneider R, Randolph GW, Barczynski M, et al. Continuous intraoperative neural monitoring of the recurrent nerves in thyroid surgery: a quantum leap in technology. Gland Surg 2016;5:607-16. [Crossref] [PubMed]
- Schneider R, Przybyl J, Hermann M, et al. A new anchor electrode design for continuous neuromonitoring of the recurrent laryngeal nerve by vagal nerve stimulations. Langenbecks Arch Surg 2009;394:903-10. [Crossref] [PubMed]
- Liddy W, Barber SR, Cinquepalmi M, et al. The electrophysiology of thyroid surgery: electrophysiologic and muscular responses with stimulation of the vagus nerve, recurrent laryngeal nerve, and external branch of the superior laryngeal nerve. Laryngoscope 2017;127:764-71. [Crossref] [PubMed]
- Brauckhoff K, Vik R, Sandvik L, et al. Impact of EMG changes in continuous vagal nerve monitoring in high-risk endocrine neck surgery. World J Surg 2016;40:672-80. [Crossref] [PubMed]
- Terris DJ, Chaung K, Duke WS. Continuous vagal nerve monitoring is dangerous and should not routinely be done during thyroid surgery. World J Surg 2015;39:2471-6. [Crossref] [PubMed]
- Almquist M, Thier M, Salem F. Cardiac arrest with vagal stimulation during intraoperative nerve monitoring. Head Neck 2016;38:E2419-20. [Crossref] [PubMed]
- Schneider R, Machens A, Bucher M, et al. Continuous intraoperative monitoring of vagus and recurrent laryngeal nerve function in patients with advanced atrioventricular block. Langenbecks Arch Surg 2016;401:551-6. [Crossref] [PubMed]
- Friedrich C, Ulmer C, Rieber F, et al. Safety analysis of vagal nerve stimulation for continuous nerve monitoring during thyroid surgery. Laryngoscope 2012;122:1979-87. [Crossref] [PubMed]
- Xiaoli L, Wu CW, Kim HY, et al. Gastric acid secretion and gastrin release during continuous vagal neuromonitoring in thyroid surgery. Langenbecks Arch Surg 2017;402:265-72. [Crossref] [PubMed]
- Dionigi G, Chiang FY, Dralle H, et al. Safety of neural monitoring in thyroid surgery. Int J Surg 2013;11:S120-6. [Crossref] [PubMed]
- Wu CW, Lu IC, Randolph GW, et al. Investigation of optimal intensity and safety of electrical nerve stimulation during intraoperative neuromonitoring of the recurrent laryngeal nerve: a prospective porcine model. Head Neck 2010;32:1295-301. [Crossref] [PubMed]
- Wu CW, Randolph GW, Lu IC, et al. Intraoperative neural monitoring in thyroid surgery: lessons learned from animal studies. Gland Surg 2016;5:473-80. [Crossref] [PubMed]
- Schneider R, Przybyl J, Pliquett U, et al. A new vagal anchor electrode for real-time monitoring of the recurrent laryngeal nerve. Am J Surg 2010;199:507-14. [Crossref] [PubMed]
- Lörincz BB, Möckelmann N, Busch CJ, et al. Automatic periodic stimulation of the vagus nerve during single-incision transaxillary robotic thyroidectomy: Feasibility, safety, and first cases. Head Neck 2016;38:482-5. [Crossref] [PubMed]
- Dionigi G, Kim HY, Wu CW, et al. Neuromonitoring in endoscopic and robotic thyroidectomy. Updates Surg 2017;69:171-9. [Crossref] [PubMed]
- Snyder SK, Sigmond BR, Lairmore TC, et al. The long-term impact of routine intraoperative nerve monitoring during thyroid and parathyroid surgery. Surgery 2013;154:704-11. [Crossref] [PubMed]
- Lombardi CP, Carnassale G, Damiani G, et al. "The final countdown": Is intraoperative, intermittent neuromonitoring really useful in preventing permanent nerve palsy? Evidence from a meta-analysis. Surgery 2016;160:1693-706. [Crossref] [PubMed]
- Vasileiadis I, Karatzas T. Comment on: "The final countdown": Is intraoperative intermittent neuromonitoring (IONM) really useful in preventing permanent nerve palsy? Evidence from a meta-analysis. Surgery 2017;161:1744-5. [Crossref] [PubMed]
- Chung TK, Rosenthal EL, Porterfield JR, et al. Examining national outcomes after thyroidectomy with nerve monitoring. J Am Coll Surg 2014;219:765-70. [Crossref] [PubMed]
- Pisanu A, Porceddu G, Podda M, et al. Systematic review with meta-analysis of studies comparing intraoperative neuromonitoring of recurrent laryngeal nerves versus visualization alone during thyroidectomy. J Surg Res 2014;188:152-61. [Crossref] [PubMed]
- Deniwar A, Bhatia P, Kandil E. Electrophysiological neuromonitoring of the laryngeal nerves in thyroid and parathyroid surgery: A review. World J Exp Med 2015;5:120-3. [Crossref] [PubMed]
- Malik R, Linos D. Intraoperative neuromonitoring in thyroid surgery: a systematic review. World J Surg 2016;40:2051-8. [Crossref] [PubMed]
- Yang S, Zhou L, Lu Z, et al. Systematic review with meta-analysis of intraoperative neuromonitoring during thyroidectomy. Int J Surg 2017;39:104-13. [Crossref] [PubMed]
- Wong KP, Mak KL, Wong CK, et al. Systematic review and meta-analysis on intra-operative neuro-monitoring in high-risk thyroidectomy. Int J Surg 2017;38:21-30. [Crossref] [PubMed]
- Barczyński M, Konturek A, Cichoń S. Randomized clinical trial of visualization versus neuromonitoring of recurrent laryngeal nerves during thyroidectomy. Br J Surg 2009;96:240-6. [Crossref] [PubMed]
- Liu XL, Wu CW, Zhao YS, et al. Exclusive real-time monitoring during recurrent laryngeal nerve dissection in conventional monitored thyroidectomy. Kaohsiung J Med Sci 2016;32:135-41. [Crossref] [PubMed]
- Schneider R, Bures C, Lorenz K, et al. Evolution of nerve injury with unexpected EMG signal recovery in thyroid surgery using continuous intraoperative neuromonitoring. World J Surg 2013;37:364-8. [Crossref] [PubMed]
- Schneider R, Randolph G, Dionigi G, et al. Prospective study of vocal fold function after loss of the neuromonitoring signal in thyroid surgery: The International Neural Monitoring Study Group’s POLT Study. Laryngoscope 2016;126:1260-6. [Crossref] [PubMed]
- Schneider R, Lorenz K, Sekulla C, et al. Surgical strategy during intended total thyroidectomy after loss of EMG signal on the first side of resection. Chirurg 2015;86:154-63. [Crossref] [PubMed]
- Barczyński M, Randolph GW, Cernea CR, et al. External branch of the superior laryngeal nerve monitoring during thyroid and parathyroid surgery: International Neural Monitoring Study Group standards guideline statement. Laryngoscope 2013;123:S1-14. [Crossref] [PubMed]
- Potenza AS, Phelan EA, Cernea CR, et al. Normative intra-operative electrophysiologic waveform analysis of superior laryngeal nerve external branch and recurrent laryngeal nerve in patients undergoing thyroid surgery. World J Surg 2013;37:2336-42. [Crossref] [PubMed]
- Kandil E, Mohamed SE, Deniwar A, et al. Electrophysiologic identification and monitoring of the external branch of superior laryngeal nerve during thyroidectomy. Laryngoscope 2015;125:1996-2000. [Crossref] [PubMed]
- Sritharan N, Chase M, Kamani D, et al. The vagus nerve, recurrent laryngeal nerve, and external branch of the superior laryngeal nerve have unique latencies allowing for intraoperative documentation of intact neural function during thyroid surgery. Laryngoscope 2015;125:E84-9. [Crossref] [PubMed]
- Deniwar A, Kandil E, Randolph G. Electrophysiological neural monitoring of the laryngeal nerves in thyroid surgery: review of the current literature. Gland Surg 2015;4:368-75. [PubMed]
- Rocke DJ, Goldstein DP, de Almeida JR. A cost-utility analysis of recurrent laryngeal nerve monitoring in the setting of total thyroidectomy. JAMA Otolaryngol Head Neck Surg 2016;142:1199-205. [Crossref] [PubMed]
- Loch-Wilkinson TJ, Stalberg PL, Sidhu SB, et al. Nerve stimulation in thyroid surgery: is it really useful? ANZ J Surg 2007;77:377-80. [Crossref] [PubMed]
- Al-Qurayshi Z, Kandil E, Randolph GW. Cost-effectiveness of intraoperative nerve monitoring in avoidance of bilateral recurrent laryngeal nerve injury in patients undergoing total thyroidectomy. Br J Surg 2017;104:1523-31. [Crossref] [PubMed]
- Dionigi G, Wu CW, Kim HY, et al. Severity of recurrent laryngeal nerve injuries in thyroid surgery. World J Surg 2016;40:1373-81. [Crossref] [PubMed]