The development and validation of biomarkers-based scoring systems for predicting early recurrence in patients with borderline resectable pancreatic cancer undergoing resection after neoadjuvant therapy
Highlight box
Key findings
• Biomarkers-based perioperative scoring systems for early recurrence (ER) prediction in patients with borderline resectable pancreatic cancer (BRPC) who underwent resection after neoadjuvant therapy (NAT) were established.
What is known and what is new?
• NAT improves current treatment strategy in BRPC, however, ER frequently occurs and is associated with a poor prognosis in patients undergoing surgery after NAT.
• How to predict ER and provide risk stratification for ER in patients with BRPC undergoing NAT are essential, but it remains unclear.
What is the implication, and what should change now?
• Scoring systems predicting ER in patients with BRPC undergoing NAT will assist clinicians in improving their treatment strategy.
Introduction
Pancreatic ductal adenocarcinoma (PDAC) is among the most aggressive human malignancies (1). At diagnosis, nearly 80% of patients have unresectable tumors, including locally advanced pancreatic cancer (LAPC) and metastatic pancreatic cancer, whereas only 20% of patients with either resectable pancreatic cancer (RPC) or borderline RPC (BRPC) are surgical candidates (2). Curative resection remains the most effective approach for achieving prolonged survivals in patients with PDAC. However, recurrence is common and significantly contributes to poor overall survival (OS) in patients undergoing radical resection (3). Owing to postoperative complications and early recurrence (ER), up to 50% of patients are unable to complete standard adjuvant therapy (AT) (4). In this context, the therapeutic strategy has shifted from upfront surgery to neoadjuvant therapy (NAT) first, to achieve optimal outcomes. Emerging evidence in the past decades has demonstrated that NAT can improve long-term survivals in patients with primarily BRPC or LAPC who undergo radical resection (5,6). Besides, increasing studies have focused on potential benefits of NAT in patients with RPC (7,8).
Given that surgical technologies have advanced the boundaries for resection, resectability may not be a limitation for patients with BRPC who will undergo surgery after NAT; instead, ER is a major consideration. Recurrence is frequently observed within two years in patients with BRPC/LAPC (60–80%), even after NAT (9), and 23–49% of all recurrences occur within 6 months after surgery (10). Patients with ER have extremely poor survivals (11). In patients with BRPC who have received NAT, identifying candidates for surgery who may have less recurrence and determining whether patients are at a high risk of ER, are closely associated with patients’ long-term outcomes. Serum tumor markers including carbohydrate antigen 19-9 (CA19-9), carcinoembryonic antigen (CEA) and CA125, pathological parameters including tumor size, vascular invasion, poor tumor differentiation, lymph node metastasis and margin status, and absence of AT were the most frequently identified risk factors of ER in PDAC (12,13). Previous risk models for predicting ER in patients with PDAC, regardless of NAT, may not be suitable for this new setting where the biochemical status has already been altered after NAT. Nevertheless, very few approaches for evaluating ER unique to patients with NAT have been developed in this field. Tsuchiya et al. identified the uptake value of positron emission tomography/computed tomography (PET/CT) and microvascular invasion of tumors, as independent risk factors of ER in patients with BRPC undergoing resections after NAT, however, PET/CT is not a convenient and economical method to predict ER when patients need to undergo repeated evaluations during NAT (14). Narayanan et al. found positive lymph node ratio as a significant predictor of ER in patients with PDAC undergoing NAT and surgery, which could only be used postoperatively (10). Hwang et al. developed a nomogram based on CA19-9 levels after NAT, tumor size on imaging at diagnosis, age, symptom, and planned surgery, which included not only BRPC, but also RPC and LAPC (15).
Serum CA19-9 has been identified as a critical prognostic biomarker in PDAC (16,17). CA19-9 response to NAT has gained significant attention for its role in evaluating treatment efficacy and stratifying prognosis in NAT patients (18). Additionally, for patients who have undergone surgery, the postoperative CA19-9 response has been demonstrated to be a critical indicator of both therapeutic efficacy and prognosis (19). Accordingly, using the CA19-9 response to construct scoring systems for predicting ER, may enable clinicians to improve perioperative therapeutic strategies in NAT patients; however, there are still some limitations in relying on the CA19-9 response alone to predict ER. Whether the CA19-9 response is an independent preoperative or postoperative risk factor for recurrence is unclear, and using a single variable to stratify the risk of ER is often inaccurate. The risk of ER may differ even among patients with the same type of CA19-9 response. Therefore, a few studies have used both CA19-9 response and tumor size reduction after NAT, to predict recurrence in patients undergoing surgery (20-22). However, they are still unable to provide a reliable prediction, because morphological changes in radiology following NAT are not in parallel with the effect of NAT, due to a high rate of desmoplastic stroma in PDAC (23).
This study investigated the role of post-NAT and postoperative CA19-9 responses in predicting ER, in patients initially presenting with BRPC who underwent surgery after NAT. We aimed to develop preoperative and postoperative scoring systems based on independent risk factors for recurrence, to establish a risk stratification of ER in patients with BRPC undergoing NAT. This may help clinicians identify candidates for surgery during the NAT process and administer individualized AT postoperatively. We present this article in accordance with the TRIPOD reporting checklist (available at https://gs.amegroups.com/article/view/10.21037/gs-2024-500/rc).
Methods
Study design and patients
Patients with PDAC who underwent curative resection after NAT in the Department of Pancreatic Surgery at Huashan Hospital between January 2018 and January 2023 were retrospectively enrolled. This study was conducted in accordance with the Declaration of Helsinki and its subsequent amendments. The study was approved by the Institutional Ethics Committee of Huashan Hospital (No. 1037), and the requirement for individual consent in this retrospective analysis was waived.
The inclusion criteria were as follows: (I) a pathological diagnosis of PDAC; (II) curative-intent resection after NAT; and (III) initial presentation of BRPC. The exclusion criteria were as follows: (I) consistent serum CA19-9 level within the normal range; (II) death within 90 days after surgery caused by surgery-related complications or other reasons instead of tumors; and (III) other uncontrolled malignancies.
Treatment scheme
The resectability status was discussed at a multidisciplinary meeting according to the guidelines of the National Comprehensive Cancer Network. NAT was recommended in patients with BRPC, LAPC, or RPC who were highly suspected to have distant metastasis. NAT regimens included gemcitabine/nab-paclitaxel and mfolfirinox. Curative resection included pancreaticoduodenectomy (PD), distal pancreatectomy (DP), and total pancreatectomy (TP). Portomesenteric vein resection and vein reconstruction were performed if portomesenteric vein involvement (PVI) was observed. AT was administered one month after surgery.
Data collection
Serum tumor markers, including CA19-9, CA125, and CEA, were collected at three time points: within one week before NAT, within one week before surgery, and one month after surgery. The post-NAT CA19-9 response (comparison between pre-NAT and preoperative CA19-9 levels) was classified into two categories: CA19-9 levels decreasing to the normal range and CA19-9 levels unable to decrease to the normal range. The postoperative CA19-9 response (comparison between preoperative and postoperative CA19-9 levels) was classified into two categories: decreasing and increasing CA19-9 levels. Serum inflammation-nutrition-coagulation parameters, including neutrophil to lymphocyte ratio (NLR), platelet to lymphocyte ratio (PLR), neutrophil to albumin (ALB) ratio (NAR), and platelet to ALB ratio (PAR) were collected within one week before surgery and one month after surgery.
Perioperative clinical parameters, including preoperative parameters [NAT regimens, age, sex, preoperative body mass index (BMI), smoking, drinking, weight loss, pain, diabetes mellitus (DM), jaundice, tumor site, planned operation, contact with artery, and PVI], and postoperative parameters (complications and postoperative BMI) were recorded. Major complication (Clavien-Dindo score ≥3) was defined according to the Clavien-Dindo classification (24).
Pathological parameters including tumor site, tumor size, tumor differentiation, perineural invasion, PV invasion, margin status (R0/R1), lymph node metastasis ratio (positive nodes/total nodes), and pathological stage were retrieved. Pathological stage was determined according to the 8th edition of the American Joint Committee on Cancer. Margin status was defined according to the International Study Group of Pancreatic Surgery criteria (25).
Follow-up
Follow-ups were performed every month during the first 6 months after surgery, then every three months during the following 6 months, and every 3–6 months in the second year. Serum biochemical marker tests (including CA19-9, CEA and CA125) and imaging examinations (including contrast-enhanced CT, magnetic resonance imaging and PET/CT) were performed to evaluate recurrence or metastasis. Serum biochemical marker tests were carried out at each follow-up visit and imaging examinations were performed every three months. Recurrence was classified as ER (within 6 months after surgery) or delayed recurrence (DR) (beyond 6 months). Recurrence-free survival (RFS) was defined as the time from surgery to recurrence or the end of follow-up. OS was defined as the time from surgery to death or the end of follow-up.
Construction of prognostic scoring systems
In this study, the univariate Cox regression analysis was performed to identify candidate parameters, which were included in the multivariate Cox regression analysis to construct the model.
Preoperative variables including the post-NAT CA19-9 response, serum tumor markers, inflammation-immune-coagulation parameters, and clinical parameters, were included in the univariate Cox regression analysis. Preoperative variables with a P<0.05 in the univariate Cox regression analysis were included in the multivariate Cox regression analysis. Variables with a P<0.05 in the multivariate Cox regression analysis were identified as independent risk factors for recurrence, which were used to construct a preoperative prognostic nomogram (R package: rms). The post-NAT prognostic score (PNPS) was calculated according to the following formula based on the coefficients in the Cox regression model: PNPS = coefficient1 × variable1 + coefficient2 × variable2 + … + coefficientN × variableN.
Postoperative variables, including the postoperative CA19-9 response, clinical parameters, pathological parameters, and serum biochemical parameters, were investigated using univariate and multivariate Cox regression analyses. Independent postoperative variables were used to establish a postoperative prognostic nomogram (R package: rms). The postoperative prognostic score (PPS) was calculated based on the corresponding coefficients in the Cox regression model as follows: PPS = coefficient1 × variable1 + coefficient2 × variable2 + … + coefficientN × variableN.
Discrimination and calibration were performed to assess the performances of the prediction models. The PNPS or PPS was used to distinguish high-risk patients from low-risk patients based on the cut-off value (R package: survminer). A calibration curve was plotted to evaluate the agreement between the observed RFS and model-predicted RFS (R package: rms). Internal validation of the model was performed using the bootstrapping method (R package: rms, repetition =500). Bootstrapping allows for validation without splitting the dataset, which is particularly useful for small datasets and leads to more stable and less biased results. Each bootstrap sample is created by randomly selecting observations with replacement. For each bootstrap sample, it trains the model using the same algorithm and parameters, followed by validating the model using the original dataset.
Statistical analysis
Receiver operating characteristic (ROC) curve analysis was performed to evaluate the accuracy of ER prediction (R package: timeROC and pROC). The area under the curve (AUC) was then calculated. The cut-off value was determined using Youden’s index (Table S1). Decision curve analysis (DCA) was performed to evaluate the net benefit of a scoring system by weighing the risk and benefit. Survival analysis was performed using the Kaplan-Meier survival plot and log-rank test (R package: survival and survminer).
For this retrospective study, the sample size was determined based on the actual available cases, according to the inclusion and exclusion criteria. The rule of the sample size for constructing the model, was to ensure at least 10 events for each candidate parameters (26). Missing data were handled using the multiple imputation method (R package: mice). Continuous variables with normal distribution were presented as mean ± standard deviation (SD), whereas variables with non-normal distribution were presented as median and interquartile range (IQR). Categorical variables were presented as absolute numbers and percentages. Continuous variables were compared using the Student’s t-test or Wilcoxon test, as appropriate, whereas categorical variables were compared using Pearson’s Chi-squared test or Fisher’s exact test, as appropriate. P<0.05 was considered statistically significant in two-tailed tests. Statistical analyses were performed using SPSS software (version 26.0; SPSS Inc., Chicago, IL, USA) and R software (version 4.3.3).
Results
Cohort characteristics
A total of 251 patients with PDAC, underwent surgery after NAT between January 2018 and January 2023 at our center. According to the inclusion and exclusion criteria, 57 patients were excluded from the cohort, including six patients initially presenting with RPC, 29 patients initially presenting with LAPC, 10 patients whose serum CA19-9 levels were consistently within the normal range, five patients who died within 90 days postoperatively, and seven patients who could not be followed up. In total, 194 patients were enrolled in this study. No radiotherapy was recorded in this cohort during NAT. All the patients underwent AT after surgery. The median follow-up time was 15.53 months (IQR, 9.48–23.06 months), and recurrence occurred in 69.0% of patients during the follow-up. ER accounted for 61.1% of all recurrences, of which hepatic metastasis (45.3%), indeterminate sites (28%), and peritoneal recurrence (12%) ranked among the top three. Patients with ER had both poorer RFS (P<0.001) and OS (P<0.001) than those with DR. Cohort characteristics are summarized in Table 1. The missing data included Pre_CA125 (1 case), Post_ALB (3 cases), Post_NLR (1 case), Post_PLR (1 case), Post_NAR (3 cases), Post_PAR (3 cases), Post_CA19-9 (3 cases), Post_CEA (2 cases) and Post_CA125 (2 cases), were handled using the multiple imputation method.
Table 1
Characteristics | Overall cohort (n=194) |
---|---|
NAT regimensa | 144 (74.2) |
Post-NAT CA19-9 responseb | 64 (32.9) |
Sex (male) | 105 (54.1) |
Age, years | 58.5 (53.0–66.0) |
Pre_BMI, kg/m2 | 22.31±2.85 |
Weight loss | 24 (12.3) |
Pain | 132 (68.0) |
Jaundice | 31 (15.9) |
DM | 52 (26.8) |
Smoking | 14 (7.2) |
Drinking | 12 (6.1) |
Operation typec | 113 (58.2) |
PVI | 113 (58.2) |
Contact with artery | 82 (42.2) |
Major complication | 4 (2.0) |
Tumor sited | 112 (57.7) |
Poor differentiation | 96 (49.4) |
Tumor size, cm | 3.0 (2.5–4.0) |
Lymph node metastasis ratio (%) | 3.6 (0.0–12.9) |
Perineural invasion | 157 (80.9) |
PV invasion | 36 (18.5) |
Margin status (R0/R1) | 133/61 |
Pathological stage (stage III) | 113 (58.2) |
Post_BMI, kg/m2 | 21.15±2.66 |
Postoperative CA19-9 responsee | 86 (44.3) |
Data are presented as n (%), median (IQR), mean ± SD, or number. a, gemcitabine/nab-paclitaxel; b, decreasing to the normal range; c, pancreaticoduodenectomy and total pancreatectomy; d, head or neck of pancreas; e, a decreasing postoperative CA19-9 level compared to preoperative CA19-9. BMI, body mass index; CA19-9, carbohydrate antigen 19-9; DM, diabetes mellitus; IQR, interquartile range; NAT, neoadjuvant therapy; PV, portomesenteric vein; PVI, portomesenteric vein involvement; SD, standard deviation.
Construction of the preoperative scoring system
Univariate and multivariate Cox regression analyses of the preoperative variables are presented in Table 2. Four variables with a P<0.05 in univariate Cox regression analysis, including post-NAT CA19-9 response, preoperative CA19-9 level, preoperative CEA level, and preoperative CA125 level, were investigated in the multivariate Cox regression analysis. Preoperative CA19-9 and CA125 levels were identified as independent risk factors for recurrence.
Table 2
Variables | Univariate analysis | Multivariate analysis | |||
---|---|---|---|---|---|
HR (95% CI) | P value | HR (95% CI) | P value | ||
NAT regimensa | 1.075 (0.647–1.786) | 0.78 | |||
Post-NAT CA19-9 responseb | 0.631 (0.422–0.944) | 0.02 | 0.762 (0.501–1.160) | 0.20 | |
Sex (male) | 1.136 (0.787–1.640) | 0.49 | |||
Age | 0.995 (0.975–1.015) | 0.63 | |||
Pre_BMI | 1.024 (0.957–1.094) | 0.48 | |||
Weight loss | 0.822 (0.461–1.465) | 0.50 | |||
Pain | 1.152 (0.776–1.709) | 0.48 | |||
Jaundice | 1.244 (0.781–1.982) | 0.35 | |||
DM | 1.356 (0.908–2.025) | 0.13 | |||
Smoking | 1.140 (0.576–2.255) | 0.70 | |||
Drinking | 0.608 (0.267–1.386) | 0.23 | |||
Pre_ALB | 0.992 (0.938–1.050) | 0.80 | |||
Pre_NLR | 1.019 (0.953–1.089) | 0.57 | |||
Pre_PLR | 1.000 (0.997–1.002) | 0.92 | |||
Pre_NAR | 0.932 (0.021–39.706) | 0.97 | |||
Pre_PAR | 0.980 (0.897–1.071) | 0.66 | |||
Pre_CA19-9 | 1.000 (1.0004–1.0008) | <0.001 | 1.0005 (1.0003–1.001) | <0.001 | |
Pre_CEA | 1.013 (1.000–1.026) | 0.04 | 1.006 (0.991–1.021) | 0.42 | |
Pre_CA125 | 1.020 (1.010–1.030) | <0.001 | 1.013 (1.002–1.025) | 0.02 | |
Operation typec | 1.173 (0.802–1.716) | 0.41 | |||
PVI | 1.210 (0.838–1.748) | 0.30 | |||
Contact with artery | 1.019 (0.703–1.475) | 0.92 |
a, gemcitabine/nab-paclitaxel; b, decreasing to the normal range; c, pancreaticoduodenectomy and total pancreatectomy. ALB, albumin; BMI, body mass index; CA125, carbohydrate antigen 125; CA19-9, carbohydrate antigen 19-9; CEA, carcinoembryonic antigen; CI, confidence interval; DM, diabetes mellitus; HR, hazard ratio; NAR, neutrophil to albumin ratio; NAT, neoadjuvant therapy; NLR, neutrophil to lymphocyte ratio; PAR, platelet to albumin ratio; PLR, platelet to lymphocyte ratio; PVI, portomesenteric vein involvement.
The independent risk factors were used to construct a preoperative prognostic nomogram (Figure 1A). The PNPS was calculated according to the formula: PNPS = 0.0006 × Pre_CA199 + 0.0144 × Pre_CA125. The calibration curve for the preoperative prediction model is shown in Figure 1B. The internal validation of the preoperative prediction model is shown in Table S2.
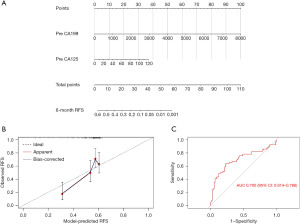
Preoperative scoring system predicting ER
ROC curve analysis was performed to investigate the accuracy of PNPS in predicting ER. The AUC of PNPS in predicting ER was 0.700 (95% CI: 0.614–0.786) (Figure 1C). The cut-off value of PNPS was 0.35886. The specificity and sensitivity of this preoperative scoring system for distinguishing high-risk patients from low-risk patients, were 86.4% and 48.7%, respectively.
Stratified analysis of prognosis based on the PNPS
The cohort was categorized into the high-risk (PNPS ≥0.35886) and low-risk (PNPS <0.35886) groups according to the PNPS cut-off value. The Kaplan-Meier survival plot analysis of RFS stratified by the PNPS is shown in Figure 2A. The high-risk group (median: 2.93 months, IQR, 1.91–5.97 months) had a significantly poorer RFS compared with the low-risk group (median: 6.90 months, IQR, 3.62–13.6 months) (P<0.001). The Kaplan-Meier survival plot analysis of OS stratified by the PNPS is shown in Figure 2B. The high-risk group (median: 7.61 months, IQR, 5.63–11.79 months) had a significantly poorer OS compared with the low-risk group (median: 12.73 months, IQR, 7.90–20.10 months) (P<0.001). The recurrence types stratified by the PNPS are shown in Figure 2C. The high-risk group had a higher ER ratio than the low-risk group (75.0% vs. 30.9%, P<0.001).
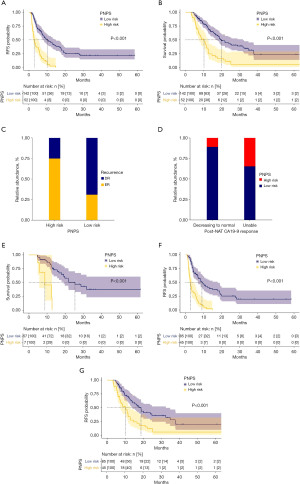
The ratios of high-risk (PNPS ≥0.35886) and low-risk (PNPS <0.35886) cases stratified by the post-NAT CA19-9 response are presented in Figure 2D. The group with a preoperative CA19-9 level decreasing to the normal range had a lower ratio of high-risk cases compared with those without (10.9% vs. 34.6%, P<0.001). In the group with a preoperative CA19-9 level decreasing to the normal range, 10.9% were at high risk and had a significantly poorer OS (P<0.001) compared to those at low risk (Figure 2E). Notably, in the group with a preoperative CA19-9 level unable to decrease to the normal range, 65.3% were at low risk and had both better RFS (P<0.001) and OS (P<0.001) than those at high risk (Figure 2F,2G).
Construction of the postoperative scoring system
Univariate and multivariate Cox regression analyses of the postoperative variables are shown in Table 3. Eight variables with a P<0.05, including poor differentiation, tumor size, lymph node metastasis ratio, tumor-node-metastasis (TNM) stage III, postoperative ALB, postoperative CA19-9, postoperative CA125, and postoperative CA19-9 response, in the univariate Cox regression analysis, were investigated in the multivariate Cox regression analysis. Four variables, including poor differentiation, tumor size, postoperative CA19-9 level, and postoperative CA19-9 response, were identified as independent risk factors for recurrence.
Table 3
Variables | Univariate analysis | Multivariate analysis | |||
---|---|---|---|---|---|
HR (95% CI) | P value | HR (95% CI) | P value | ||
Major complication | 0.848 (0.269–2.672) | 0.77 | |||
Tumor sitea | 1.124 (0.771–1.639) | 0.54 | |||
Poor differentiation | 1.629 (1.118–2.374) | 0.01 | 1.665 (1.038–2.671) | 0.03 | |
Tumor size | 1.241 (1.116–1.379) | <0.001 | 1.227 (1.070–1.406) | 0.003 | |
Lymph node metastasis ratio | 4.035 (1.446–11.261) | 0.007 | 1.129 (0.351–3.625) | 0.83 | |
Perineural invasion | 1.365 (0.833–2.236) | 0.21 | |||
PV invasion | 1.141 (0.740–1.759) | 0.54 | |||
Margin status (R1) | 1.391 (0.950–2.037) | 0.08 | |||
TNM stage III | 1.805 (1.232–2.646) | 0.002 | 1.303 (0.810–2.096) | 0.27 | |
Post_BMI | 1.038 (0.954–1.130) | 0.38 | |||
Post_ALB | 0.935 (0.893–0.979) | 0.004 | 0.958 (0.912–1.006) | 0.09 | |
Post_NLR | 1.000 (0.906–1.103) | >0.99 | |||
Post_PLR | 0.999 (0.996–1.001) | 0.43 | |||
Post_NAR | 1.599 (0.180–14.161) | 0.67 | |||
Post_PAR | 1.005 (0.949–1.065) | 0.84 | |||
Post_CA19-9 | 1.000 (1.0001–1.0003) | <0.001 | 1.0002(1.0000–1.0004) | 0.04 | |
Post_CEA | 1.003 (0.999–1.007) | 0.10 | |||
Post_CA125 | 1.002 (1.000–1.004) | 0.01 | 0.998 (0.994–1.001) | 0.31 | |
Postoperative CA19-9 responseb | 0.609 (0.410–0.905) | 0.01 | 0.526 (0.336–0.822) | 0.004 |
a, head or neck of pancreas; b, a decreasing postoperative CA19-9 level compared to preoperative CA19-9. ALB, albumin; BMI, body mass index; CA125, carbohydrate antigen 125; CA19-9, carbohydrate antigen 19-9; CEA, carcinoembryonic antigen; CI, confidence interval; HR, hazard ratio; NAR, neutrophil to albumin ratio; NLR, neutrophil to lymphocyte ratio; PAR, platelet to albumin ratio; PLR, platelet to lymphocyte ratio; PV, portomesenteric vein; TNM, tumor-node-metastasis.
The independent risk factors were used to construct a postoperative prognostic nomogram (Figure 3A). The PPS was calculated using the following formula: PPS = 0.6404 × Poor_differentiation + 0.1679 × Tumor_size + 0.0002 × Post_CA19-9 − 0.6314 × Post_CA199_response. The calibration curve of the postoperative prediction model is shown in Figure 3B. The internal validation of the postoperative prediction model is presented in Table S3.
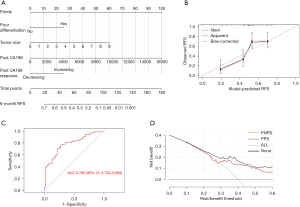
Postoperative scoring system predicting ER
ROC curve analysis was performed to investigate the accuracy of PPS in predicting ER. The AUC of PPS in predicting ER was 0.785 (95% CI: 0.705–0.866) (Figure 3C). The cut-off value of PPS was 0.43949. The specificity and sensitivity of this postoperative scoring system for distinguishing high-risk patients from low-risk patients, were 65.4% and 80.8%, respectively.
The net benefits of both preoperative and postoperative scoring systems with different risk/benefit thresholds were showed (Figure 3D). It assumed that the reasonable risk/benefit thresholds were 0.25 and 0.33, indicating that underestimating the high risk of ER would be three and two times worse respectively, than the unnecessary intervention due to a false prediction of ER. For the preoperative scoring system, the net benefits were 0.182 and 0.158 when the risk/benefit thresholds were 0.25 and 0.33, respectively, equivalent to that 18 patients and 15 patients per 100 patients would be identified as high-risk patients respectively, and really benefit from this scoring system. For the postoperative scoring system, the net benefits were 0.223 and 0.190 when the risk/benefit thresholds were 0.25 and 0.33, respectively, equivalent to that 22 patients and 19 patients per 100 patients would be identified as high-risk patients respectively, and really benefit from this scoring system.
Stratified analysis of prognosis based on the PPS
The cohort was categorized into the high-risk (PPS ≥0.43949) and low-risk (PPS <0.43949) groups according to the PPS cut-off value. The Kaplan-Meier survival plot analysis of RFS stratified by the PPS is shown in Figure 4A. The high-risk group (median: 4.23 months, IQR, 2.40–7.56 months) had a significantly poorer RFS compared with the low-risk group (median: 7.66 months, IQR, 4.66–17.80 months) (P<0.001). The Kaplan-Meier survival plot analysis of OS stratified by the PPS is presented in Figure 4B. The high-risk group (median: 9.63 months, IQR, 6.53–15.33 months) had a significantly poorer OS than the low-risk group (median: 14.03 months, IQR, 8.16–28.83 months) (P<0.001). The recurrence types stratified by the PPS are presented in Figure 4C. The high-risk group had a higher ratio of ER compared with the low-risk group (55.5% vs. 23.3%, P<0.001).
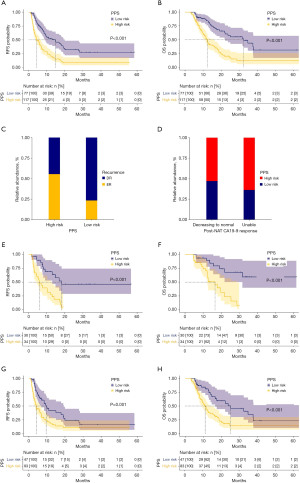
The ratios of high-risk (PPS ≥0.43949) and low-risk (PPS <0.43949) cases stratified by the post-NAT CA19-9 response are presented in Figure 4D. No significant difference in the ratios of high-risk cases was identified between the group with a preoperative CA19-9 level decreasing to the normal range and those without (53.1% vs. 63.8%, P=0.20). Notably, in the group with a preoperative CA19-9 level decreasing to the normal range, 53.1% were at high risk and had both poorer RFS (P<0.001) and OS (P<0.001) compared with those at low risk (Figure 4E,4F). In the group with a preoperative CA19-9 level that did not decrease to the normal range, 36.1% were at low risk and had both better RFS (P=0.002) and OS (P<0.001) than those at high risk (Figure 4G,4H).
Discussion
The present evidence suggests the application of NAT in patients with BRPC, LAPC, or even RPC (27). The main goals of NAT are to eliminate occult micro-metastatic lesions, increase the ratio of R0 resection, reduce tumor volume and the rate of lymph node metastasis, identify candidates for curative surgery by ruling out patients with early metastasis during the NAT period, and evaluate chemosensitivity and tolerance to treatment (27). In BRPC, advanced disease and vessel involvement frequently lead to ER after surgery, even in patients who have undergone NAT; however, identifying patients who are at a high risk of ER remains unclear.
In this study, although the post-NAT CA19-9 response was identified as a significant risk factor for recurrence in the univariate analysis, it was not an independent risk factor in the multivariate analysis. The preoperative prognostic nomogram used independent preoperative risk factors for recurrence including preoperative CA19-9 and CA125 levels to predict ER. CA19-9 and CA125 are classic serum tumor markers and risk factors for the prognosis of PDAC (16,28). Using this nomogram, clinicians can predict ER probability for individuals who have undergone NAT, whereas relying on the post-NAT CA19-9 response alone may not provide ER prediction for individuals (18). Based on this scoring system, clinicians can perform ER risk stratification in patients undergoing NAT. Patients with PNPS ≥0.35886 are considered to be at high risk, and have both poorer RFS and OS compared with those at low risk. This indicates that extended NAT or enhanced AT may be therapeutic options for high-risk patients.
The optimal cutoff for defining the post-NAT CA19-9 response, whether based on a decrease to the normal range or by any ratios, remains inconclusive (29). Different approaches of classifying post-NAT CA19-9 responses may generate different risk stratifications in the same group of patients, although each approach has prognostic value. This suggests that using cut-off values to classify the CA19-9 response cannot further improve the accuracy of stratifying prognosis. In patients whose serum CA19-9 levels decreased to the normal range after NAT, a subgroup (10.9%) was identified at the high risk of ER (PNPS ≥0.35886), who had poorer OS compared with those at low risk (PNPS <0.35886). Notably, in patients whose serum CA19-9 levels did not normalize after NAT, a subgroup (65.3%) was identified at the low risk of ER (PNPS <0.35886), who had both better RFS and OS compared with those at high risk (PNPS ≥0.35886). This highlights the inaccuracy of relying solely on the post-NAT CA19-9 response to stratify prognosis. Such an approach may result in some patients with good post-NAT CA19-9 responses to have poor prognosis after surgery, or some patients without ideal post-NAT CA19-9 responses to miss the best timing for surgery. Our preoperative scoring system may help clinicians in identifying patients without an ideal post-NAT CA19-9 response who are actually at a low risk of ER, or patients with a good post-NAT CA19-9 response who are at a high risk of ER. This can enhance the decision-making process before surgery, including selecting candidates for surgery and determining the optimal timing for surgery.
Preoperative evaluation can help clinicians in identifying candidates for surgery, whereas postoperative prediction of prognosis promotes individualized management of patients who have undergone surgery after NAT. Our postoperative scoring system can predict ER based on tumor differentiation, tumor size, postoperative CA19-9 level, and postoperative CA19-9 response. The postoperative CA19-9 level is a critical prognostic marker in patients undergoing surgery (30,31). The dynamic change in postoperative CA19-9 levels has been used to evaluate the response to AT and monitor recurrence (19,32). We used the change in postoperative CA19-9 levels as a response to surgery, which may indicate both the curative effect of surgery after NAT, and occult active tumors after surgery. The use of postoperative CA19-9 response to predict ER is the main feature of this system, which increases the accuracy of predicting ER compared with the preoperative scoring system. Clinicians can stratify ER based on the PPS. Patients with PPS ≥0.43949 were at high risk and have both poorer RFS and OS than those with PPS <0.43949. High-risk patients are more likely to experience ER; therefore, this subgroup requires close follow-up. Even if patients with good post-NAT CA19-9 responses benefit from radical surgery, 53.1% of them remain at a high risk of ER. Clinicians can identify these high-risk patients using our postoperative scoring system at a very early stage after surgery, and administer AT with enhanced regimens or for an extended duration to improve outcomes.
The median AUC of previous factors or models for predicting ER in PDAC was 0.646 (IQR, 0.615–0.681), while the specificity and sensitivity were 65.2% (IQR, 64.7–65.5%) and 57.3% (IQR, 55.2–59.8%) respectively (12,33-36). AUCs of previous models for predicting ER in patients undergoing NAT were 0.736 (15) and 0.662 (14). The AUCs of preoperative and postoperative scoring systems in this study were 0.700 and 0.785, respectively, which were both within a reasonable range. The preoperative scoring system in this study with high specificity (86.4%) and relatively low sensitivity (48.7%) may identify truly high-risk patients before surgery, which minimizes the possible risk of unnecessary surgery delays due to a false prediction of ER. The postoperative scoring system in this study with relatively low specificity (65.4%) and high sensitivity (80.8%) may provide early warnings for all high-risk patients after surgery, which minimizes the possible risk of underestimating ER during the process of AT or follow-up. The DCA in this study further validated the significance of applying both preoperative and postoperative scoring systems in real-world clinical practice.
There are some limitations in this study. First, this was a retrospective study with inevitable bias. Second, the relatively small sample size restricted grouping and stratified analysis. Third, the regimen and duration of NAT, surgical procedures and techniques, and management of patients in a single center may affect the interpretation of the results, which should be verified in a multi-center study. Finally, the dynamic changes in other biochemical markers after NAT or surgery warrant further investigation. Scoring systems, including changes in multiple biochemical markers after NAT or surgery, may further improve the accuracy of ER prediction, which will be our future research direction.
Conclusions
This study has established preoperative and postoperative scoring systems that provide approaches for predicting ER in patients with BRPC undergoing surgery after NAT. The preoperative system allows clinicians to predict the probability of ER in patients undergoing NAT and provides a more precise stratification of prognosis, contributing to the decision-making process before surgery, including selecting candidates for surgery and the timing of surgery. The postoperative scoring system has been developed based on the postoperative CA19-9 response, further increasing the accuracy of predicting ER to identify patients undergoing surgery after NAT who are actually at a high risk of ER. Through this postoperative system, clinicians can administer AT with an enhanced regimen or an extended duration to prevent or delay recurrence in high-risk patients. Both the preoperative and postoperative systems in this study will help improve treatment strategies for patients with BRPC who undergo surgery after NAT.
Acknowledgments
None.
Footnote
Reporting Checklist: The authors have completed the TRIPOD reporting checklist. Available at https://gs.amegroups.com/article/view/10.21037/gs-2024-500/rc
Data Sharing Statement: Available at https://gs.amegroups.com/article/view/10.21037/gs-2024-500/dss
Peer Review File: Available at https://gs.amegroups.com/article/view/10.21037/gs-2024-500/prf
Funding: This work was supported by
Conflicts of Interest: All authors have completed the ICMJE uniform disclosure form (available at https://gs.amegroups.com/article/view/10.21037/gs-2024-500/coif). The authors have no conflicts of interest to declare.
Ethical Statement: The authors are accountable for all aspects of the work in ensuring that questions related to the accuracy or integrity of any part of the work are appropriately investigated and resolved. The study was conducted in accordance with the Declaration of Helsinki and its subsequent amendments. The study was approved by the institutional ethics committee of Huashan Hospital (No. 1037) and individual consent for this retrospective analysis was waived.
Open Access Statement: This is an Open Access article distributed in accordance with the Creative Commons Attribution-NonCommercial-NoDerivs 4.0 International License (CC BY-NC-ND 4.0), which permits the non-commercial replication and distribution of the article with the strict proviso that no changes or edits are made and the original work is properly cited (including links to both the formal publication through the relevant DOI and the license). See: https://creativecommons.org/licenses/by-nc-nd/4.0/.
References
- Siegel RL, Giaquinto AN, Jemal A. Cancer statistics, 2024. CA Cancer J Clin 2024;74:12-49. [Crossref] [PubMed]
- Mizrahi JD, Surana R, Valle JW, et al. Pancreatic cancer. Lancet 2020;395:2008-20. [Crossref] [PubMed]
- Groot VP, Rezaee N, Wu W, et al. Patterns, Timing, and Predictors of Recurrence Following Pancreatectomy for Pancreatic Ductal Adenocarcinoma. Ann Surg 2018;267:936-45. [Crossref] [PubMed]
- Merkow RP, Bilimoria KY, Tomlinson JS, et al. Postoperative complications reduce adjuvant chemotherapy use in resectable pancreatic cancer. Ann Surg 2014;260:372-7. [Crossref] [PubMed]
- Ghaneh P, Palmer D, Cicconi S, et al. Immediate surgery compared with short-course neoadjuvant gemcitabine plus capecitabine, FOLFIRINOX, or chemoradiotherapy in patients with borderline resectable pancreatic cancer (ESPAC5): a four-arm, multicentre, randomised, phase 2 trial. Lancet Gastroenterol Hepatol 2023;8:157-68. [Crossref] [PubMed]
- Brown ZJ, Cloyd JM. Trends in the utilization of neoadjuvant therapy for pancreatic ductal adenocarcinoma. J Surg Oncol 2021;123:1432-40. [Crossref] [PubMed]
- Birrer DL, Golcher H, Casadei R, et al. Neoadjuvant Therapy for Resectable Pancreatic Cancer: A New Standard of Care. Pooled Data From 3 Randomized Controlled Trials. Ann Surg 2021;274:713-20. [Crossref] [PubMed]
- Endo Y, Kitago M, Kitagawa Y. Evidence and Future Perspectives for Neoadjuvant Therapy for Resectable and Borderline Resectable Pancreatic Cancer: A Scoping Review. Cancers (Basel) 2024;16:1632. [Crossref] [PubMed]
- Cass SH, Tzeng CD, Prakash LR, et al. Trends Over Time in Recurrence Patterns and Survival Outcomes after Neoadjuvant Therapy and Surgery for Pancreatic Cancer. Ann Surg 2024; Epub ahead of print. [Crossref] [PubMed]
- Narayanan S, AlMasri S, Zenati M, et al. Predictors of early recurrence following neoadjuvant chemotherapy and surgical resection for localized pancreatic adenocarcinoma. J Surg Oncol 2021;124:308-16. [Crossref] [PubMed]
- Daamen LA, Dorland G, Brada LJH, et al. Preoperative predictors for early and very early disease recurrence in patients undergoing resection of pancreatic ductal adenocarcinoma. HPB (Oxford) 2022;24:535-46. [Crossref] [PubMed]
- Murakawa M, Kawahara S, Takahashi D, et al. Risk factors for early recurrence in patients with pancreatic ductal adenocarcinoma who underwent curative resection. World J Surg Oncol 2023;21:263. [Crossref] [PubMed]
- Leonhardt CS, Gustorff C, Klaiber U, et al. Prognostic Factors for Early Recurrence After Resection of Pancreatic Cancer: A Systematic Review and Meta-Analysis. Gastroenterology 2024;167:977-92. [Crossref] [PubMed]
- Tsuchiya N, Matsuyama R, Murakami T, et al. Risk Factors Associated With Early Recurrence of Borderline Resectable Pancreatic Ductal Adenocarcinoma After Neoadjuvant Chemoradiation Therapy and Curative Resection. Anticancer Res 2019;39:4431-40. [Crossref] [PubMed]
- Hwang HK, Wada K, Kim HY, et al. A nomogram to preoperatively predict 1-year disease-specific survival in resected pancreatic cancer following neoadjuvant chemoradiation therapy. Chin J Cancer Res 2020;32:105-14. [Crossref] [PubMed]
- van Oosten AF, Groot VP, Dorland G, et al. Dynamics of Serum CA19-9 in Patients Undergoing Pancreatic Cancer Resection. Ann Surg 2024;279:493-500. [Crossref] [PubMed]
- Zou WB, Zhang XP, Zhao ZM, et al. Recognizing significance of propensity score matching and clinical practice in evaluating oncological survivals of pancreatic cancer. Hepatobiliary Surg Nutr 2025;14:179-80. [Crossref] [PubMed]
- Newhook TE, Vreeland TJ, Griffin JF, et al. Prognosis Associated With CA19-9 Response Dynamics and Normalization During Neoadjuvant Therapy in Resected Pancreatic Adenocarcinoma. Ann Surg 2023;277:484-90. [Crossref] [PubMed]
- Imamura H, Tomimaru Y, Kobayashi S, et al. Diagnostic impact of postoperative CA19-9 dynamics on pancreatic cancer recurrence: a single-institution retrospective study. Updates Surg 2024;76:479-86. [Crossref] [PubMed]
- Maggino L, Malleo G, Crippa S, et al. CA19.9 Response and Tumor Size Predict Recurrence Following Post-neoadjuvant Pancreatectomy in Initially Resectable and Borderline Resectable Pancreatic Ductal Adenocarcinoma. Ann Surg Oncol 2023;30:207-19. [Crossref] [PubMed]
- Terai T, Nagai M, Nakamura K, et al. Combination of carbohydrate antigen 19-9 level and tumor size after neoadjuvant chemoradiation therapy may predict early recurrence of resectable pancreatic ductal adenocarcinoma. Pancreatology 2023;23:970-7. [Crossref] [PubMed]
- Servin-Rojas M, Fong ZV, Fernandez-Del Castillo C, et al. Tumor Size Reduction and Serum Carbohydrate Antigen 19-9 Kinetics After Neoadjuvant FOLFIRINOX in Patients With Pancreatic Ductal Adenocarcinoma. Surgery 2024;175:471-6. [Crossref] [PubMed]
- Wang ZJ, Arif-Tiwari H, Zaheer A, et al. Therapeutic response assessment in pancreatic ductal adenocarcinoma: society of abdominal radiology review paper on the role of morphological and functional imaging techniques. Abdom Radiol (NY) 2020;45:4273-89. [Crossref] [PubMed]
- DeOliveira ML, Winter JM, Schafer M, et al. Assessment of complications after pancreatic surgery: A novel grading system applied to 633 patients undergoing pancreaticoduodenectomy. Ann Surg 2006;244:931-7; discussion 937-9. [Crossref] [PubMed]
- Bockhorn M, Uzunoglu FG, Adham M, et al. Borderline resectable pancreatic cancer: a consensus statement by the International Study Group of Pancreatic Surgery (ISGPS). Surgery 2014;155:977-88. [Crossref] [PubMed]
- Riley RD, Ensor J, Snell KIE, et al. Calculating the sample size required for developing a clinical prediction model. BMJ 2020;368:m441. [Crossref] [PubMed]
- Manojlovic N, Savic G, Manojlovic S. Neoadjuvant treatment of pancreatic ductal adenocarcinoma: Whom, when and how. World J Gastrointest Surg 2024;16:1223-30. [Crossref] [PubMed]
- Luo X, Lin X, Lin R, et al. The CA125 level postoperative change rule and its prognostic significance in patients with resectable pancreatic cancer. BMC Cancer 2023;23:832. [Crossref] [PubMed]
- Ye C, Sadula A, Ren S, et al. The prognostic value of CA19-9 response after neoadjuvant therapy in patients with pancreatic cancer: a systematic review and pooled analysis. Cancer Chemother Pharmacol 2020;86:731-40. [Crossref] [PubMed]
- Kondo N, Murakami Y, Uemura K, et al. Comparison of the prognostic impact of pre- and post-operative CA19-9, SPan-1, and DUPAN-II levels in patients with pancreatic carcinoma. Pancreatology 2017;17:95-102. [Crossref] [PubMed]
- Rieser CJ, Zenati M, Hamad A, et al. CA19-9 on Postoperative Surveillance in Pancreatic Ductal Adenocarcinoma: Predicting Recurrence and Changing Prognosis over Time. Ann Surg Oncol 2018;25:3483-91. [Crossref] [PubMed]
- Xu HX, Li S, Wu CT, et al. Postoperative serum CA19-9, CEA and CA125 predicts the response to adjuvant chemoradiotherapy following radical resection in pancreatic adenocarcinoma. Pancreatology 2018;18:671-7. [Crossref] [PubMed]
- Hong S, Song KB, Hwang DW, et al. Preoperative serum carbohydrate antigen 19-9 levels predict early recurrence after the resection of early-stage pancreatic ductal adenocarcinoma. World J Gastrointest Surg 2021;13:1423-35. [Crossref] [PubMed]
- Liu W, Tang B, Wang F, et al. Predicting early recurrence for resected pancreatic ductal adenocarcinoma: a multicenter retrospective study in China. Am J Cancer Res 2021;11:3055-69. [PubMed]
- Ishido K, Kimura N, Wakiya T, et al. Development of a Biomarker-Based Scoring System Predicting Early Recurrence of Resectable Pancreatic Duct Adenocarcinoma. Ann Surg Oncol 2022;29:1281-93. [Crossref] [PubMed]
- Li S, Zhang G, Lu Y, et al. Perioperative Serum Scoring Systems Predict Early Recurrence and Poor Prognosis of Resectable Pancreatic Cancer. Front Oncol 2022;12:841819. [Crossref] [PubMed]