Continuous intraoperative neural monitoring of the recurrent nerves in thyroid surgery: a quantum leap in technology
Principles of neural monitoring in thyroid surgery
Routine dissection and identification of the recurrent laryngeal nerve (RLN) in thyroid surgery are proven as safe and valuable methods of protecting it from operative injury (1). RLN visualization is considered the gold standard for prevention of RLN injury during thyroid surgery (2). It is recognized that the leading source of postoperative RLN dysfunction is not transection but it is nonstructural functional nerve injury (3-6). Since the introduction of intraoperative neural monitoring (IONM) in thyroid surgery, more than 50 years ago (7), the technology has considerably improved during the past decade (8-11). The use of intermittent stimulation of the RLN and the vagus nerve has now become a standard practice in numerous specialized centers around the globe. Modern nerve monitoring, whether intermittent [intermittent nerve monitoring (IIONM)] or continuous [continuous intraoperative neural monitoring (CIONM)], helps to identify the nerve, maps its course during thyroid dissection, diagnoses segmental (type 1) and diffuse (type 2) loss of signal (LOS), and offers basis for change of surgical strategy in the setting of LOS of the first side in a planned bilateral thyroid surgery (12).
The main advantage of CIONM using temporary implantable vagus electrode compared to IIONM is the real-time surveillance of RLN function during thyroid mobilization and RLN dissection, which IIONM cannot offer. The substantial drawback of the IIONM format using the handheld stimulation probe is consequent to its limitation that exposes the RLN to the risk of injury in-between two stimulations. Therefore, functional impairment of the nerve can only be detected after the damage has already occurred. Thus IIONM does not provide an opportunity to take the critical surgical action necessary to avert the eventual RLN injury (11).
CIONM, the newer format of IONM, overcomes this limitation of IIONM; by offering a real-time uninterrupted RLN monitoring; thereby allowing recognition of impending nerve injury and appreciation of intraoperative recovery of nerve function after loss of electromyographic (EMG) signal (13). At present, various types of electrodes with diverse designs are available and are distinguishable based on the extent of dissection required for positioning them on the vagus nerve (14). It is important to notice that in the acronym CIONM the term “continuous” refers to the word “monitoring” emphasizing the constant nature of the monitoring throughout the surgery without the need to interrupt the operation once the vagal electrode is positioned for nerve stimulation. CIONM at the outset may seem unreasonable and can be viewed as excessive non-physiological nerve stimulation. It is a well-known principle of neurophysiology that continuous stimulation of a peripheral motor nerve leads to a single contraction of the innervated muscle. CIONM may be more accurately described as repeated pulsed stimulation that is contemporaneous with the surgical maneuvers (11). The possible advantage of a CIONM format is that it has the potential to monitor the entire vagus and RLN functional integrity in real-time throughout surgery and could identify EMG signals associated with early-impending injury states (8,9,11,15-18). As shown by Schneider et al. (19) the final outcome of RLN dysfunction was significantly improved by CIONM. In this series with 965 nerves at risk using IIONM and 1,314 nerves at risk using CIONM permanent vocal cord palsy (VCP) rate could be diminished from 0.4% to 0% (P=0.019).
Safety of continuous neural monitoring
Beside two anecdotal reports of local and cardiac complications during CIONM in a very few patients (20,21), there are several clinical data showing no evidence of adversity related to the circular dissection of the a vagal nerve segment or related intrinsically to CIONM (11,15,17,19,22-27).
It is of utmost importance to realize that 1 to 2 mA current, used in the CIONM technique do not activate thin demyelinated C-fibers responsible for the greatest autonomic effects (28). The stimulation with 1 mA is supramaximal for only the efferent motor A fibers and myelinated autonomic B fibers. These findings are consistent with the animal data, demonstrating that the amplitude of the elicited EMG signal cannot be augmented by increasing the stimulation current above a threshold of 0.7 to 0.8 mA (9,29). Low level vagus nerve stimulation at frequencies less than 30 Hz (maximum frequency in CIONM is 3 Hz) has not been associated with subsequent adverse vagal effects. Furthermore, the 1 mA stimulation current used for CIONM is not believed to engender concomitant or subsequent adverse vagal effect leading to central (headache, numbness), cardiac (arrhythmias, bradycardia), pulmonary (bronchospasm), or gastrointestinal (nausea, vomiting) symptoms (9,11,15,17,26,27,30,31). Studies (22,25) that applied stimulation current up to 5.0 mA for CIONM, observed an increased variability in heart rate, implying autonomic nervous system imbalance. The elicited parasympathetic activation was not associated with a compensatory increase in sympathetic activity and remained subclinical.
If the standards of IONM including gentle vagus nerve and RLN dissection are applied stringently, there is no evidence that CIONM intrinsically causes harm. Moreover, even older patients with advanced AV block and/or pacemaker can be monitored safely by CIONM (32). Reports of safe application of vagal electrode in single incision transaxillary robotic thyroid surgery and for minimally invasive esophagectomy are also found in the literature (33,34).
Operating instructions for continuous neural monitoring
CIONM technique generally comprises multichannel EMG system, EMG display, sensing endotracheal surface electrode, handheld stimulation electrode, and is complemented by additional temporarily placed vagus electrode (35,36). Synchronic use of both the handheld stimulation probe for identification and tracking the RLN course, and vagus electrode for real-time scrutiny of RLN function during CIONM is proposed.
All manufactures (Medtronic, Jacksonville, USA; Inomed, Emmendingen, Germany; Dr. Langer Medical, Waldkirch, Germany) provide neuromonitoring devices with varying EMG display, alarm limit setting, and vagal electrode configurations.
The succeeding points should be considered for the safe and reliable use of vagus electrode:
- Surgeries: cervicotomy ranging from minimal invasive to extended Kocher incision;
- Access to the vagus nerve within the carotid sheath before exposing the thyroid by anterior (midline) or lateral (between sternohyoid and sternocleidomastoid muscle) approach: the advantage of midline incision is the use in small skin incisions, like for benign thyroid surgery. The alternative lateral approach employed especially in huge thyroid goiter, revision procedures and thyroid cancer surgery has the advantage of lower rate of incidental electrode dislocation because the electrical wire is outside the operative field;
- Vagus nerve stimulation by hand held stimulation probe to test the integrity and function of the nerve prior to the CIONM electrode placement: primarily negative vagus nerve stimulation response should be clarified using the troubleshooting algorithm as for IONM (12). The right vagus nerve should be stimulated inferiorly to confirm or exclude non-recurrent inferior laryngeal nerve with an incidence less than 1.0%;
- Circular (360°) gentle dissection of a small vagal nerve segment (in contrast to IIONM): it is of fundamental importance to avoid nerve devascularization during its isolation and mobilization;
- Initial stimulation: by 1 mA and 1 Hz;
- System calibration with highest achievable amplitude: it is established that the amplitude of the so called “baseline” reference curve must be ≥500 µV during initial calibration of the CIONM system to guarantee a stable and reliable EMG signal (11). Maximum amplitude values are valuable for two reasons. Firstly, sufficient amplitude is the prerequisite for the stable and reliable calculation of latency. Secondly, the higher the amplitude, the wider is the tolerance for dissection related maneuvers heralding impending nerve injury;
- Baseline at the start of CIONM: once the reference curve (“baseline”) of the CIONM is obtained; changes in it (amplitude and latency) are displayed on a timeline. It is possible to set an audible and visual alert when threshold values are exceeded, to help the surgeon identify risky maneuvers. Exposing the thyroid gland, RLN identification and its dissection are identical to that of IIONM, using the handheld stimulation probe (12).
Recognition of impending nerve injury by continuous neural monitoring
The diagnosis of LOS implies the complete loss of neural stimulation response with amplitude ≤100 µV, as defined by the International Neuromonitoring Study Group (INMSG) (12).
The skilful use of CIONM requires experience and observation of the EMG screen to quickly respond to abnormal EMG signal and alarms (37). Meaningful warning thresholds need to be defined and implemented such that automated alarms are sounded and visually displayed once a critical EMG threshold is exceeded. Isolated amplitude decrease to less than 50% from baseline or latency increase to less than 110% of baseline may be artefactual, believed to arise from tube malrotation or tracheal shifting with impaired contact between the recording endotracheal tube surface electrodes and vocal cord as a result of thyroid manipulation and traction or be considered “subclincal adverse EMG events” (11). Besides temporary loss of EMG recording caused by bipolar forceps coagulation, dislocation of the vagus electrode may occur after inadvertent pulling on the conduction wire; or a mismatch between size of the vagus nerve and the electrode may result in poor stimulation characteristics or prompt the electrode to detach.
In contrast to the non-dangerous artefacts (Figure 1A-C), which typically resolve after repositioning of the thyroid into its original position, dangerous traction related EMG changes, not resolving after thyroid repositioning, need to be differentiated (Figure 1D-F). The EMG changes during CIONM are classified into non-dangerous changes, combined events and LOS based on their relative risk for RLN injury (Table 1). In adverse EMG changes, the percent change in amplitude and latency differed quantitatively. Based on animal studies with experimental RLN injury induced by applying direct traction, pressure and heat on the RLN altered the EMG signal in a reproducible characteristic manner (9). Herein, changes in amplitude (decreases by 40–60%) were more marked than changes in latency (increases by 15%), the extent of which depended on the kind of injury. To facilitate the interpretation of clinically relevant quantitative EMG signals, adverse “combined” EMG events (CE) (Figure 1D), encompassing specific concordant changes in both signal amplitude and latency, were defined as greater than 50% decrease in amplitude along with greater than 10% increase in latency regarding to baseline values demonstrating clinically important EMG thresholds indicative of impending nerve injury (11). It has been established in a proof of concept study with 52 patients (and 52 nerves at risk) that inappropriate traction on the thyroid can induce stretch injury of the RLN two points, (I) where RLN courses through the ligament of Berry; (II) or at the point where the RLN intersects the inferior thyroid artery (11). Medial retraction of the thyroid lobe has been shown to reduce the amplitude by more than 60%, which can be restored after the reversing the related surgical maneuver. Traction on the laryngotracheal angle, leading to compromise of the RLN at its laryngeal entry site, as well as lobar retraction distant from RLN can trigger CE. In another series of 102 patients, severe CE was defined as amplitude decrease to less than 70% of baseline amplitude and increase in latency to more than 10% of baseline latency (17). Intraoperative severe CE was associated with development of VCP (P=0.001). CE had a PPV of 33%, NPV of 97%, and was reversible in 73%. Isolated amplitude or latency changes were not associated with VCP. Recently, it was shown in 788 patients (1,314 nerves at risk) with CIONM guided thyroid surgery that immediate reaction by withdrawing nerve traction did not proceed to LOS in 80% (n=63 of 77) of CE (19). This electrophysiological correlate of impending nerve dysfunction may occur in two phases of neuroanatomical changes (Figure 2). The first phase represents amplitude decrease as a reduction of the number of neurofibers transmitting action potential due to RLN traction. The second phase with further amplitude decrease and latency increase could be translated as an additional increase of time interval to vocal muscle action by continued RLN traction while the number of myofibers has already been reduced. Our understanding of these possible phases is still nascent as the trauma to the RLN in these human cases is speculative and not quantified. We expect that there could be different initial and more engaged phases in impending RLN neuropraxic injury but this will likely require additional animal model work. Figure 3 illustrates two examples of circumvention of second phase of CE by immediate discontinuation of surgical traction of the thyroid.
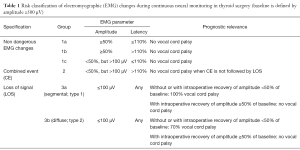
Full table
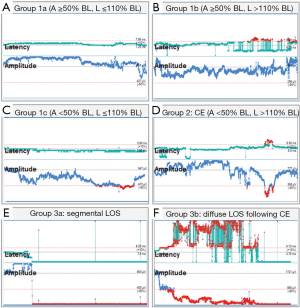
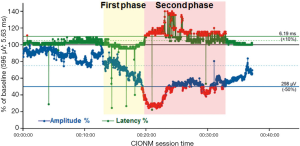
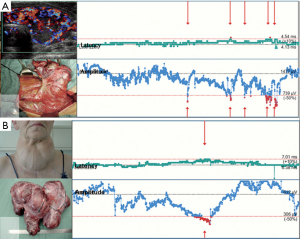
Our progressive understanding of CE during our five year experience with CIONM has shown that right at the onset of CE, RLN traction should be immediately released by repositioning the thyroid and then one needs to wait for the amplitude increase back to more than 50% of the baseline amplitude. If traction-related repeated decrease of amplitude below 50% of baseline occurs several times, the inferior/lateral/or superior approach to the thyroid lobe should be altered to medial dissection to the thyroid hilum to prevent further dangerous EMG changes. In contrast, if CE allowed to last, it can advance to LOS (which typically is significantly less reversible) and to postoperative VCP (Figure 1F) (11,13,17).
Recognition of intraoperative recovery of nerve function after LOS by continuous neural monitoring
Segmental RLN lesions are caused by direct prompt trauma to the nerve such as nerve transection, clamping, ligation, pinching, or bipolar coagulation. Diffuse nerve lesions might be the result of more indirect global forces, subsequent to more global traction on the airway or tissues adjacent to the nerve (6) The segmental and diffuse nerve injuries differ greatly in terms of the time to loss of the nerve monitoring signal as well as in the dynamics of loss of the nerve amplitude (medians of 2 vs. 156 seconds; P log-rank <0.001) (19). Unlike most mechanical trauma, thermal or transection injuries in which the nerve monitoring signal was lost all on a sudden (Figure 1E), RLN traction injuries were always preceded by CE (Figure 1F). Because segmental LOS are less likely to result in graded partial EMG changes which can be identified before definitive injury has occurred, CIONM might not help to prevent this type of lesion, but might weaken the severity of nerve injury through sudden reaction by the operating surgeon. Again the onset of adverse EMG changes in type 1 or type 2 is likely importantly related to the type, magnitude and time course of the trauma associated with it. It is likely these largely unknown elements that are major determinants of the secondary associated adverse EMG events. Advanced training and careful microdissection are instrumental in minimizing these injuries, since certain anatomic settings such as thin nerves, extralaryngeal branching, and nerves coursing anterior to the inferior thyroid artery increase the risk of segmental nerve injuries (11,18). In contrast, RLN traction injuries may be prevented by CIONM as traction may result in CE, prompting modification of the underlying surgical maneuver (11). As most recently shown by an international multicenter study of 115 LOS cases, 4/5th (n=92/115) of LOS are caused by traction of the RLN (6).
The INMSG definition of LOS with EMG change from initial satisfactory EMG to no or low (≤100 µV) response with stimulation at 1 to 2 mA in a dry field and no laryngeal twitch (12) has its limitation in pre-existing VCP with 14% of patients having intact EMG (38) and after intraoperative recovery of EMG amplitude after LOS (13). As recently shown nerve recovery after segmental or diffuse injury is diagnosed when amplitude increases from ≤100 µV back to >100 µV. There are two different types of intraoperative recovery:
- Incomplete recovery (consistent with postoperative VCP): amplitude increases to >100 µV but <50% of baseline (Figure 4A);
- Complete recovery (consistent with postop intact VC function): amplitude increase to ≥50% of baseline (Figure 4B) (13).
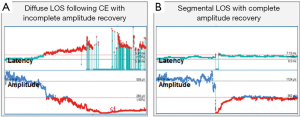
The analysis of 18 nerves at risk with LOS and intraoperative EMG recovery showed a correlation of degree of intraoperative amplitude recovery (% of baseline) after LOS with postop VCP. The lower the percentage of amplitude recovery (30% vs. 40% vs. 50%) relative to the baseline amplitude the higher the percentage of postoperative VCP (36% vs. 22% vs. 0%) can be calculated (unpublished data).
As recently shown in literature, nerve function completely recovered after LOS within the first 20 min (segmental RLN lesion: 6.9 min; diffuse RLN lesion: 15.6 min), which means decision making should be done after 20 minutes of waiting time (13). If the amplitude did not recover or recovers to only <50% of baseline, it is not advisable to wait longer than 20 minutes, since it is known that all (n=16/16) patients with segmental nerve injury or three quarter (n=13/18) of patients with diffuse lesion had impaired early vocal cord function. Noticeably, the severity of vocal cord dysfunction correlated with the severity of nerve dysfunction. Three fifth (n=10/16) of patients with severe segmental nerve injury without or incomplete EMG recovery showed complete VCP, whereas only 1/3 (n=4/13) patients with diffuse RLN lesion showed complete vocal cord dysfunction, indicating less severe damage.
Intraoperative change of strategy when using continuous neural monitoring
Only strict adherence to L1, V1, R1, R2, V2, L2 approach published by INMSG is set to improve the reliability of neural monitoring (12). CIONM has reliability that is superior to IIONM (19), with sensitivity of 90.9%, specificity of 99.7%, PPV of 88.2%, and NPV of 99.8%. It enables the possibility to modify the surgical plan after LOS and correctly performed troubleshooting algorithm for LOS (Figure 5). An injured nerve, independent of type of LOS, requires a minimum wait of 20 minutes upon LOS to recover ≥50% of its baseline amplitude before moving on with completion thyroidectomy on the unaffected side (13). If the nerve amplitude fails to recover at least 50% of its baseline amplitude after 20 minutes, there is 85.3% (n=29/34 patients) risk of early postoperative VCP. These data force the surgeon to reconsider the surgical plan, especially in the setting of LOS on the first side of resection in a planned bilateral surgery. Staged thyroidectomy—not before 2–3 months after initial surgery, but after recovery of vocal cord function—should be drawn as the logical approach after recovery of vocal cord function (39,40). As shown in the literature, diffuse RLN lesions had faster time to recovery of vocal cord function compared to segmental lesions (medians of 27 vs. 62 days; P<0.01) (13). In absence of complete intraoperative recovery of amplitude, contralateral surgery should only performed in exceptional circumstances, which has to be discussed with the patient preoperatively as an individual decision.
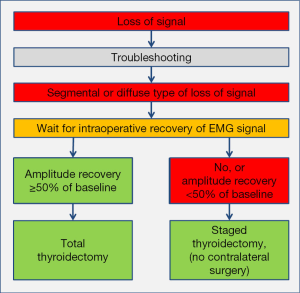
CIONM’s predictive accuracy is very high with 99.5%, and represents a perfect basis for intraoperative decision making in favour of or against contralateral surgery. The lower rates of false positive and false negative with CIONM compared to IIONM (0.3% vs. 0.5%; 0.2% vs. 0.6%; n.s.) may further decrease the number of unnecessary staged procedures in false positive, and of potential bilateral VCP in false negative findings, in particular when the concept of incomplete and complete amplitude recovery after LOS is incorporated in decision making in CIONM guided thyroidectomy (13,19).
Conclusions
The CIONM technique is undoubtedly an excellent adjuvant for the surgeon during routine thyroid surgery, providing a useful tool to prevent harmful manoeuvres. In the case of especially complex cases such as recurrent disease with numerous adhesions, tumors infiltrating adjacent structures, massive goiters with mediastinal invasion or tracheal deviation, continuous monitoring changes from a simple support during traditional surgical procedures to an indispensable tool that assists the surgeon during risky and complex procedures. In these circumstances, early identification of the RLN and its pathway is not always possible, despite that, once the vagal electrode is positioned the EMG obtained during the CIONM alerts the team constantly and in real time about the safety of every step.
The CE event is a pathognomonic EMG sign of impending nerve dysfunction that may progress to diffuse LOS, for which CIONM is the best warning system available at present that prompts immediate relief of nerve tension. In contrast, direct injury leading to segmental RLN injury with sudden drop of decrease in amplitude without concomitant latency changes, however, still can only be avoided by meticulous nerve dissection, and not by IONM.
CIONM can immediately spot RLN injury in almost real time as it were, prompting instant release of the nerve so that it has the opportunity to recover intraoperatively or weaken the severity of injury. As an advanced innovation, CIONM allows for early corrective action before permanent damage to the nerve sets in. Once LOS has been confirmed, a 20-minute wait period will allow the surgeon to know whether the affected nerve will recover fully or not and whether a staged thyroid surgery should be considered after the completion of the first side resection.
With the 5 years of experience with CIONM, permanent VCP rate is lower with CIONM and accuracy in predicting intact or non-intact early postoperative vocal cord function is superior to IIONM. Also, procedure-related false positive (unnecessary staged thyroidectomy) and false negative (risk of bilateral VCP) results can be reduced by CIONM. Nonetheless, patients should be well informed about CIONM preoperatively and its potential consequence of staged thyroidectomy in the setting of LOS on the first side in a planned bilateral surgery should be included in the informed consent.
Acknowledgements
None.
Footnote
Conflict of Interest: Henning Dralle was remunerated by Medtronic and Inomed for giving lectures on intraoperative nerve monitoring. The other authors have no conflicts of interest to declare.
References
- Lahey FH. Routine dissection and demonstration of the recurrent laryngeal nerve in subtotal thyroidectomy. Surg Gynecol Obstet 1958;66:775-77.
- Riddell V. Thyroidectomy: prevention of bilateral recurrent nerve palsy. Results of identification of the nerve over 23 consecutive years (1946-69) with a description of an additional safety measure. Br J Surg 1970;57:1-11. [Crossref] [PubMed]
- Dralle H, Sekulla C, Haerting J, et al. Risk factors of paralysis and functional outcome after recurrent laryngeal nerve monitoring in thyroid surgery. Surgery 2004;136:1310-22. [Crossref] [PubMed]
- Hermann M, Hellebart C, Freissmuth M. Neuromonitoring in thyroid surgery: prospective evaluation of intraoperative electrophysiological responses for the prediction of recurrent laryngeal nerve injury. Ann Surg 2004;240:9-17. [Crossref] [PubMed]
- Barczyński M, Konturek A, Cichoń S. Randomized clinical trial of visualization versus neuromonitoring of recurrent laryngeal nerves during thyroidectomy. Br J Surg 2009;96:240-6. [Crossref] [PubMed]
- Schneider R, Randolph G, Dionigi G, et al. Prospective study of vocal fold function after loss of the neuromonitoring signal in thyroid surgery: The International Neural Monitoring Study Group's POLT study. Laryngoscope 2016;126:1260-6. [Crossref] [PubMed]
- Flisberg K, Lindholm T. Electrical stimulation of the human recurrent laryngeal nerve during thyroid operation. Acta Otolaryngol Suppl 1969;263:63-7. [PubMed]
- Lamadé W, Ulmer C, Seimer A, et al. A new system for continuous recurrent laryngeal nerve monitoring. Minim Invasive Ther Allied Technol 2007;16:149-54. [Crossref] [PubMed]
- Schneider R, Przybyl J, Pliquett U, et al. A new vagal anchor electrode for real-time monitoring of the recurrent laryngeal nerve. Am J Surg 2010;199:507-14. [Crossref] [PubMed]
- Lamadé W, Ulmer C, Rieber F, et al. New backstrap vagus electrode for continuous intraoperative neuromonitoring in thyroid surgery. Surg Innov 2011;18:206-13. [Crossref] [PubMed]
- Schneider R, Randolph GW, Sekulla C, et al. Continuous intraoperative vagus nerve stimulation for identification of imminent recurrent laryngeal nerve injury. Head Neck 2013;35:1591-8. [Crossref] [PubMed]
- Randolph GW, Dralle H, International Intraoperative Monitoring Study Group, et al. Electrophysiologic recurrent laryngeal nerve monitoring during thyroid and parathyroid surgery: international standards guideline statement. Laryngoscope 2011;121 Suppl 1:S1-16. [Crossref] [PubMed]
- Schneider R, Sekulla C, Machens A, et al. Dynamics of loss and recovery of the nerve monitoring signal during thyroidectomy predict early postoperative vocal fold function. Head Neck 2016;38 Suppl 1:E1144-51. [Crossref] [PubMed]
- Schneider R, Lorenz K, Machens A, et al. Continuous Intraoperative Neuromonitoring (CIONM) of the Recurrent Laryngeal Nerve. In: Randolph GW. editor. The Recurrent and Superior Laryngeal Nerves. Switzerland: Springer International Publishing, 2016:169-83.
- Schneider R, Przybyl J, Hermann M, et al. A new anchor electrode design for continuous neuromonitoring of the recurrent laryngeal nerve by vagal nerve stimulations. Langenbecks Arch Surg 2009;394:903-10. [Crossref] [PubMed]
- Schneider R, Bures C, Lorenz K, et al. Evolution of nerve injury with unexpected EMG signal recovery in thyroid surgery using continuous intraoperative neuromonitoring. World J Surg 2013;37:364-8. [Crossref] [PubMed]
- Phelan E, Schneider R, Lorenz K, et al. Continuous vagal IONM prevents recurrent laryngeal nerve paralysis by revealing initial EMG changes of impending neuropraxic injury: a prospective, multicenter study. Laryngoscope 2014;124:1498-505. [Crossref] [PubMed]
- Wu CW, Wang MH, Chen CC, et al. Loss of signal in recurrent nerve neuromonitoring: causes and management. Gland Surg 2015;4:19-26. [PubMed]
- Schneider R, Sekulla C, Machens A, et al. Postoperative vocal fold palsy in patients undergoing thyroid surgery with continuous or intermittent nerve monitoring. Br J Surg 2015;102:1380-7. [Crossref] [PubMed]
- Terris DJ, Chaung K, Duke WS. Mounting Evidence of the Potential Perils Associated with Continuous Intraoperative Neuromonitoring World J Surg 2016;40:770-1. Reply. [Crossref] [PubMed]
- Brauckhoff K, Vik R, Sandvik L, et al. Impact of EMG Changes in Continuous Vagal Nerve Monitoring in High-Risk Endocrine Neck Surgery. World J Surg 2016;40:672-80. [Crossref] [PubMed]
- Friedrich C, Ulmer C, Rieber F, et al. Safety analysis of vagal nerve stimulation for continuous nerve monitoring during thyroid surgery. Laryngoscope 2012;122:1979-87. [Crossref] [PubMed]
- Dionigi G, Chiang FY, Dralle H, et al. Safety of neural monitoring in thyroid surgery. Int J Surg 2013;11 Suppl 1:S120-6. [Crossref] [PubMed]
- Ulmer C, Koch KP, Seimer A, et al. Real-time monitoring of the recurrent laryngeal nerve: an observational clinical trial. Surgery 2008;143:359-65. [Crossref] [PubMed]
- Ulmer C, Friedrich C, Kohler A, et al. Impact of continuous intraoperative neuromonitoring on autonomic nervous system during thyroid surgery. Head Neck 2011;33:976-84. [Crossref] [PubMed]
- Van Slycke S, Gillardin JP, Brusselaers N, et al. Initial experience with S-shaped electrode for continuous vagal nerve stimulation in thyroid surgery. Langenbecks Arch Surg 2013;398:717-22. [Crossref] [PubMed]
- Mangano A, Kim HY, Wu CW, et al. Continuous intraoperative neuromonitoring in thyroid surgery: Safety analysis of 400 consecutive electrode probe placements with standardized procedures. Head Neck 2016;38 Suppl 1:E1568-74. [Crossref] [PubMed]
- Groves DA, Brown VJ. Vagal nerve stimulation: a review of its applications and potential mechanisms that mediate its clinical effects. Neurosci Biobehav Rev 2005;29:493-500. [Crossref] [PubMed]
- Wu CW, Lu IC, Randolph GW, et al. Investigation of optimal intensity and safety of electrical nerve stimulation during intraoperative neuromonitoring of the recurrent laryngeal nerve: a prospective porcine model. Head Neck 2010;32:1295-301. [Crossref] [PubMed]
- Castoro MA, Yoo PB, Hincapie JG, et al. Excitation properties of the right cervical vagus nerve in adult dogs. Exp Neurol 2011;227:62-8. [Crossref] [PubMed]
- Yoo PB, Lubock NB, Hincapie JG, et al. High-resolution measurement of electrically-evoked vagus nerve activity in the anesthetized dog. J Neural Eng 2013;10:026003. [Crossref] [PubMed]
- Schneider R, Machens A, Bucher M, et al. Continuous intraoperative monitoring of vagus and recurrent laryngeal nerve function in patients with advanced atrioventricular block. Langenbecks Arch Surg 2016;401:551-6. [Crossref] [PubMed]
- Lörincz BB, Möckelmann N, Busch CJ, et al. Automatic periodic stimulation of the vagus nerve during single-incision transaxillary robotic thyroidectomy: Feasibility, safety, and first cases. Head Neck 2016;38:482-5. [Crossref] [PubMed]
- Tsang RK, Law S. Adaptation of Continuous Intraoperative Vagus Nerve Stimulation for Monitoring of Recurrent Laryngeal Nerve During Minimally Invasive Esophagectomy. World J Surg 2016;40:137-41. [Crossref] [PubMed]
- Deniwar A, Kandil E, Randolph G. Electrophysiological neural monitoring of the laryngeal nerves in thyroid surgery: review of the current literature. Gland Surg 2015;4:368-75. [PubMed]
- Angeletti F, Musholt PB, Musholt TJ. Continuous Intraoperative Neuromonitoring in Thyroid Surgery. Surg Technol Int 2015;27:79-85. [PubMed]
- Dionigi G, Donatini G, Boni L, et al. Continuous monitoring of the recurrent laryngeal nerve in thyroid surgery: a critical appraisal. Int J Surg 2013;11 Suppl 1:S44-6. [Crossref] [PubMed]
- Lorenz K, Abuazab M, Sekulla C, et al. Results of intraoperative neuromonitoring in thyroid surgery and preoperative vocal cord paralysis. World J Surg 2014;38:582-91. [Crossref] [PubMed]
- Mihai R. Loss of the nerve monitoring signal during bilateral thyroid surgery (Br J Surg 2012; 99: 1089-1095). Br J Surg 2012;99:1096. [Crossref] [PubMed]
- Schneider R, Lorenz K, Sekulla C, et al. Surgical strategy during intended total thyroidectomy after loss of EMG signal on the first side of resection. Chirurg 2015;86:154-63. [Crossref] [PubMed]