Surgical treatments of lymphedema—a literature review on robot-assisted lymphovenous anastomosis (LVA)
Introduction
Lymphedema is a common problem, affecting approximately 200 million people worldwide with a majority of cases being secondary to other conditions that cause impaired lymphatic function, such as tumor surgery and radiation, trauma, and recurring infections (1). Primary lymphedema, attributed to genetic variations leading to the development of dysfunctional lymphatic vessels with insufficient drainage (2), constitutes less than one percent of all lymphedema cases, but is relatively more common in the pediatric population where it comprises over 90% of all cases. The true prevalence of primary lymphedema, however, is mostly unknown due to under-diagnosis (3).
In addition to visible swelling of a limb having psychosocial implications and affecting a person’s mobility (4), complications of lymphedema also include recurring infections (5,6) and an increased risk of skin malignancies, including a non-negligible risk of developing angiosarcoma (7). Due to its chronic, progressive nature, lymphedema can have devastating consequences for the affected individual and is associated with significant treatment costs (8).
Surgery targeting lymphedema dates back to the beginning of the 20th century with the Charles procedure (9) and Gillies’s early descriptions of lymph node transfers, attempting to restore the lymphatic drainage from the legs to the trunk using a flap from the ipsilateral arm (10). The lymphovenous anastomosis (LVA) was first described by Yamada in 1969, to establish an alternate path for lymph drainage (11), but it was not until the beginning of the 21st century that the idea started to gain momentum with the inception of supermicrosurgery as a concept, utilizing the subdermal venular system rather than larger caliber cutaneous veins for anastomosis to the lymphatic vessels (12). Lymphaticovenous anastomosis is, as the name suggests, a technique where venules and lymphatic vessels are anastomosed, requiring supermicrosurgical technique due to the diameter of the vessels (12). While the idea started as a shunt that bypasses the area of obstruction by draining excess lymphatic fluid into the venous system (13), recent research provides a more nuanced view of the systemic effects including normalization of lymphedema-related changes in T cell profile after LVA surgery (14).
The submillimeter size of both the lymphatic vessels and the recipient veins poses a distinct set of challenges concerning the surgical technique. The lymphatic vessels are often thin-walled giving them a tendency to collapse which can make it arduous to get the needle into the lumen. In addition to this, there is often a size mismatch between the lymphatic vessel and the venule that needs to be overcome (15). A better understanding of the condition and advances in diagnostics have improved the possibilities of providing a personalized treatment approach, allowing specific tailoring of the intervention to the individual. However, the delicate nature inherent in microsurgical lymphedema treatment necessitates further advancements in improving the surgeon’s technical proficiency. The introduction of robotics in surgery has shown promising results in taking the next technological step to proceed past the confinements of the human capacity.
After the inception of the da Vinci® Surgical System (Intuitive Surgical, Inc., Sunnyvale, CA, USA) in 2000, several robotic surgery systems have been developed, with indications in general, thoracic, urologic, gynecologic, and head and neck surgery (16). Soon after their introduction, traditional robotic systems were proven to be useful in various ways in microsurgical reconstructions (17-21), with benefits being motion scaling, tremor elimination, and articulating instruments. A drawback, however, has been the lack of specialized microsurgical instruments, as the instruments of the da Vinci system can be too large and too powerful for the most delicate microsurgical tasks. In addition to this, the resolution at high levels of magnification can be insufficient for microsurgical procedures (22).
During the last decade, significant advances have been made in the field of microsurgery, and the establishment of supermicrosurgery as a concept, commonly defined as surgery on vessels smaller than 0.8 mm in diameter (22,23), has brought us closer to the limits of the capacity of the unaided human hand. Using robot assistance has shown promising results in pushing the boundaries of what is considered possible with the goal of transcending the limitations of human dexterity. Considering the nature of LVA surgery, with anastomosis often being done on a supermicrosurgical level, a wider implementation of robot assistance might make complex lymphatic surgery available to a larger proportion of patients suffering from lymphedema. This review aims to provide a structured update on the robot-assisted LVA. We present this article in accordance with the Narrative Review reporting checklist (available at https://gs.amegroups.com/article/view/10.21037/gs-24-22/rc).
Methods
A search was performed to identify all published papers to date, describing the use of robotics in LVA surgery, using the PubMed, Cochrane, and Embase databases. See Tables 1,2 for details on the search strategy. Titles and abstracts were reviewed and tested against the inclusion criteria before the included articles were fully reviewed. The inclusion criteria used were:
- Studies reporting on the use of robotic assistance in LVA surgery.
- Publication in English.
- Only original publications were included, and thus commentary and correspondence were excluded. All study designs were included.
Table 1
Items | Specification |
---|---|
Date of search | 2023-12-22 |
Databases and other sources searched | PubMed, Cochrane, Embase |
Search terms used | Lymphedema, lymphatic vessel, lymphovenous anastomosis, LVA, robotic surgical procedures, microsurgery, supermicrosurgery |
Timeframe | All publications until 2023-12-22 |
Inclusion and exclusion criteria | Studies reporting on the use of robotic assistance in LVA surgery |
Publication in English | |
Only original publications were included, and thus commentary and correspondence were excluded. All study designs were included | |
Selection process | Selection was performed by the first author. Titles and abstract were reviewed for all 65 publications. A total of 10 publications were selected for full-text review. A total of 5 publications were selected after consideration of inclusion and exclusion criteria |
LVA, lymphovenous anastomosis.
Table 2
Search | Terms and fields | Results |
---|---|---|
1 | Robotic Surgical Procedures[MeSH] OR Robotics[MeSH] OR Robot*[Title/Abstract] | 80,602 |
2 | Anastomosis, Surgical[MeSH] OR Microsurgery[MeSH] OR Anastomos*[Title/Abstract] OR Microsurgery[Title/Abstract] OR Microsurgical[Title/Abstract] OR Supramicrosurgery[Title/Abstract] OR Supermicrosurgery[Title/Abstract] OR Supramicrosurgical[Title/Abstract] OR Supermicrosurgical[Title/Abstract] OR Surgery[Title/Abstract] OR Surgical[Title/Abstract] | 2,357,837 |
3 | Lymphatic Vessel[MeSH] OR “Lymphatic Vessel*”[Title/Abstract] OR “Lymph vessel*”[Title/Abstract] Lymphovenous[Title/Abstract] OR LVA[Title/Abstract] | 20,731 |
4 | 1 AND 2 AND 3 | 34 |
5 | Lymphedema[MeSH] OR Lymphedema*[Title/Abstract] | 17,982 |
6 | 4 AND 5 | 7 |
Similar search strategies were applied to Cochrane and Embase.
Data on variables being studied and main findings, as reported by the authors, were extracted, as well as data on anastomosis time and patency when available. Quantitative statistics were not performed due to the heterogenicity of the study designs and outcomes reported.
Results
A total of 65 studies were identified through the search and after review of titles and abstracts, 10 publications were selected for full-text review. A total of five publications were found to match the inclusion criteria (Tables 3,4).
Table 3
Reference | Year | Location | Number of participants | Study design | Operations performed | Outcomes reported |
---|---|---|---|---|---|---|
van Mulken et al. (22) | 2020 | Maastricht, Eindoven, The Netherlands | 20 patients | Prospective randomized pilot study | Robot-assisted and manual supermicrosurgical LVA, due to breast cancer related lymphedema | Patient outcomes at 1- and 3-month post-surgery, duration of surgery, quality of anastomosis |
Lindenblatt et al. (24) | 2022 | Zurich, Switzerland | 5 patients | Case series | Robot-assisted and hand-sewn LVA, LLA, and free vascularized lymph node transfer, due to primary and secondary lymphedema. Tumor resections, and perforator flap reconstruction were also performed | Operation time. Number of robot-assisted and manual LVA and arterial anastomosis. Anastomosis patency |
Barbon et al. (25) | 2022 | Zurich, Switzerland | 22 patients | Retrospective study | Lymphatic reconstructive surgery, free flap reconstruction, nerve coaptation | Main focus on operating times and learning curve |
van Mulken et al. (26) | 2022 | Maastricht, Eindhoven, The Netherlands | 20 patients | Prospective randomized pilot study | Robot-assisted and manual LVA, due to breast cancer related lymphedema | 1-year follow-up. QoL, arm circumference, use of conservative measures, dermal backflow stage, anastomosis patency |
Weinzierl et al. (27) | 2023 | Zurich, Switzerland | 8 patients | Case series | LVA and omental flap transfer to the axilla, due to primary and secondary lymphedema due to breast cancer treatment | Anastomosis time and patency. Comments on ergonomics, suture precision. Case based patient outcomes |
LVA, lymphovenous anastomosis; LLA, lympho-lymphatic anastomosis; QoL, quality of life.
Table 4
Reference | Number of anastomosis | Anastomosis time | Anastomosis quality and patency | Other main findings |
---|---|---|---|---|
van Mulken et al. (22) | 40 total, 14 using robot, 26 manual |
Mean anastomosis times were significantly longer in the robot-assisted group compared with the manual group [25±6 min, range 16–33 min and 9±6 min, range 4–36 min, respectively (P<0.001)]. A steep decline in time needed for anastomosis was seen in the robot-assisted group. Mean time for the full procedure was 81 and 115 min in the manual and the robot-assisted group, respectively | Quality is evaluated using SAMS and UWOMSA. The manual group showed higher mean scores in both SAMS (4.0±0.3 vs. 3.2±0.4, P<0.001) and UWOMSA (4.0±0.5 vs. 3.4±0.3, P<0.001). Anastomosis patency was 100% at the time of surgery | Similar quality of life improvement and decrease in arm volume at 3 months in both groups. There was a decrease in Lymph-ICF scores in both the manual and the robot-assisted groups between baseline and 3 months [mean percentage −41.17 in the robot-assisted group vs. −41.57 in the manual LVA group (P=0.98)]. No difference in Lymph-ICF scores, between the manual and robot-assisted groups at 1 month (8.31, 95% CI: −6.75 to 23.37, P=0.2) or at 3 months (0.69, 95% CI: −13.41 to 14.51, P=0.92), using linear mixed model analysis |
UEL index slightly decreased in the robot-assisted group and increased in the manual group (−0.93 and 0.36, respectively, P=0.66) between baseline and 3 months. No significant difference in UEL index was seen between the manual and robot-assisted groups at 1 month (−3.95, 95% CI: −10.62 to 2.75, P=0.230) and 3 months (−0.33, 95% CI: −6.69 to 6.03, P=0.914), using a linear mixed model analysis | ||||
Two cases of erysipelas were reported in the robot-assisted group, but no serious adverse events were seen in either group | ||||
Lindenblatt et al. (24) | 10 robot-assisted anastomosis | The robot-assisted anastomosis took initially two to three times longer than the manual anastomosis. A rapid decline was seen with an increasing number of anastomosis being performed. Anastomosis times were not specified | Anastomosis patency was 100% at the time of surgery | The authors conclude that using the robotic system is feasible and safe in the context of lymphatic surgery. Adverse events are not commented on |
Barbon et al. (25) | 32 anastomosis, 20 LVA | Anastomosis times were shorter in the manual group compared to the robot-assisted group [14.1±4.3 vs. 25.3±12.3 min (P<0.01)] | Anastomosis patency was 97.5% at the time of surgery. One thrombosis was seen in the robot-assisted group | Longer anastomosis times in the robot group, however, there was a steep learning curve leading to comparable times by the end of the study. No adverse events specific to the microsurgical procedures were reported. Four seromas after tumor resection were noted |
The time needed for performing LVAs decreased between the first and the second group of patients [23.9±6.8 vs. 16.3±6.1 min (P<0.05)] | ||||
van Mulken et al. (26) | 14 robot, 24 manual | No specific times for anastomosis are specified. The mean total time of the operations was 81 (48–140) min in the manual group and 115 (69–185) min in the robot-assisted group | 66.6% of patients in the robot-assisted group and 81.8% in the manual group had at least one patent anastomosis at 12 months follow-up | Mean decrease in Lymph-ICF was 20 (38 to 18, P=0.045) points in the robot-assisted group and 23 (48 to 25, P=0.001) points in the manual group at 12 months. The mean difference in UEL index between the affected and unaffected arm was 20.6 to 23.37 (P=0.140) in the robot-assisted group and 19.71 to 23.02 (P=0.313) in the manual group. No significant intervention effect (manual vs. robot-assisted) was seen at any time point regarding Lymph-ICF or UEL index of the affected arm. Use of compression garments decreased by 42.9% in the robot-assisted group and 45.5% in the manual group. Mean frequency of manual lymphatic drainage increased in the robot-assisted group (3.69 to 3.75 times per month) and decreased in the manual group (6.5 to 2.92 times per month). Improvement in dermal backflow stage was more common in the manual group than in the robot-assisted group. There were no adverse events |
Weinzierl et al. (27) | Not specified | Average anastomosis time was 22.6±26.2 min. The first anastomosis time was 59 min, as compared with and average of 20.0±2.8 min in the remaining 7 patients | 100% patency at the time of surgery | Initially, longer anastomosis times using the robot, but a rapid decrease in time with increasing number of cases performed. Favorable ergonomics and good ease of reach in difficult-to-access locations |
SAMS, Structured Assessment of Microsurgery Skills; UWOMSA, University of Western Ontario Microsurgical Skills Acquisition Instrument; Lymph-ICF, Lymphedema Functioning, Disability, and Health questionnaire; LVA, lymphovenous anastomosis; CI, confidence interval; UEL, upper extremity lymphedema.
Discussion
In the first-in-human study on robot-assisted supermicrosurgical LVA, van Mulken et al. present a comparison between robot-assisted anastomosis and manual anastomosis. Outcome at 1 and 3 months was measured as well as surgery time and anastomosis quality. The surgery time was significantly longer using the robot system (25 vs. 9 min, P<0.001), however, the time to completion rapidly decreased in the robot group. Quality of life improved in both groups, and there were no major adverse events in any of the groups. The authors conclude that the method is safe and feasible using the MUSA system (MicroSure, Eindhoven, The Netherlands), but also note that further studies are needed. On 1-year follow-up they found that clinical outcomes, including quality of life improvement, arm circumference and decrease in daily use of compression garments were comparable between the groups, confirming the feasibility of the robot-assisted LVA (26). In another clinical study, Lindenblatt et al. report the first-in-human use of the Symani Surgical System (Medical Microinstruments, Calici, Italy) for LVA and arterial anastomosis in lymphatic reconstruction. Ten robot-assisted anastomoses were performed, noting longer times for finishing individual anastomoses, but a 100% patency and a high accuracy in placing small stitches in the most fragile tissues (24). In a case series by Weinzierl et al., the Symani system is used for LVA and omental flap transfer, and the use of a robot system with an articulated arm is concluded to be beneficial when performing surgical procedures, such as anastomoses, within a deep plane and a confined space (27). Barbon et al. focus on the learning curve of the Symani Surgical System in performing robot-assisted anastomosis for lymphatic and free flap reconstructions, as well as for nerve coaptation. Although robot-assisted times were significantly slower initially, the learning curve was found to be steep, leading to a rapid decrease in anastomosis time. By the last operations, the times to perform LVA using the robot were comparable to the time needed by hand (25).
Notably, all of the included studies have at least one author that has functioned as a consultant or clinical advisor for the company producing the robotic system used in the publication.
A robot system designed as an aid in surgical procedures needs to address certain limitations of human performance. First, performing surgery on smaller structures requires smaller, more precise movements. Using robot assistance to scale the movements of the surgeon allows for an improvement in precision in performing the most intricate tasks (26). Secondly, the human hand has a physiologic tremor of 0.5–3 mm (28) which will limit the ability to perform delicate and small supermicrosurgical tasks by hand. Although today trained supermicrosurgeons routinely perform manual anastomosis even smaller than 0.3 mm, the tremor of the human hand can never be eliminated, which is why tremor filtration is another essential aspect of the robot system (26), especially when approaching vessels of supermicrosurgical caliber. Moreover, the benefit of a robotic system has also been described when performing surgery in hard-to-reach locations of the body such as the axilla, where it might be impractical to utilize an assistant to cut sutures. Combined robotic instruments such as a needle holder that also has a cutting function can make the surgeon independent from the assistant and eliminate the need for frequent instrument changes (27). The increased reach and maneuverability in difficult locations can have implications for thoracic duct and central lymphatic system surgery. Furthermore, the benefits of improved ergonomics should not be overlooked, considering the long operating times associated with many microsurgical procedures.
As of 2023, there are two robotic systems available that were specifically developed for reconstructive plastic surgery (27).
The MUSA is designed to stabilize and scale movements as well as to filter out tremors. The system consists of two robotic arms attached to a suspension ring, that in turn attaches to the operating table (Figure 1). The robotic arms are equipped with genuine microsurgical instruments and are controlled with two forceps-like joysticks. Foot pedals are used for activating and deactivating the system, and for adjusting the motion scaling. The system is placed between the microscope and the patient, thus allowing the surgeon to use a readily available microscope (22). Suggested benefits include the fact that actual microsurgical instruments are used with the system, allowing for easier customization and access to more delicate instruments.
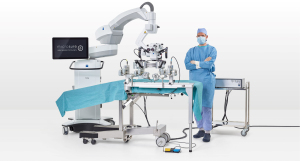
The Symani Surgical System is also based on the idea of motion scaling and tremor filtration and consists of two robotic arms equipped with microsurgical instruments with a 3 mm wrist, offering seven degrees of freedom. The arms are mounted on a free-standing suspension device and are controlled from a console composed of a chair, a foot pedal for activation and deactivation, and forceps-like joysticks (Figure 2). The system has been used with both a standard operating microscope and a 3D visualization system (24). Suggested benefits include the articulated instrument allowing better maneuverability in hard-to-reach areas.
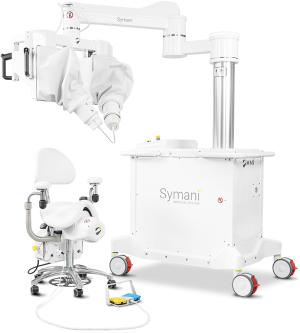
One obvious potential drawback of a robotic system is the cost. Not only the price of acquiring and maintaining the system must be taken into account, but also the costs of implementation including training and potential adjustments that have to be made to the operation rooms (27). When performing a deep inferior epigastric perforator (DIEP) flap using the da Vinci system to aid the dissection, Gundlapalli et al. reported a 10% increase in the total patient charge ($16,300 vs. $14,800) compared to the average for non-robotic cases (29). The cost of robot use per procedure is of course dependent on the case volume and an increased number of procedures being performed with robot aid will decrease the cost per procedure (30). A study on the use of surgical robots in England revealed a difference in the annual cost of maintenance and disposables per number of procedures between low-volume and high-volume centers. The total cost was almost 5.5 times higher in a low-volume center compared with the same cost in a high-volume center (£1,587 per procedure in 446 procedures vs. £8,679 per procedure in 53 procedures) (31), suggesting the potential for cost optimization in high-volume centers.
Several authors note that operating times are longer when using robot assistance but that the times significantly decrease with each procedure the surgeon performs (18,22,24,27,32), to the degree that it can be comparable to the time needed to perform the procedure by hand (25). Previous microsurgical expertise seems to be beneficial in the skill acquisition in robot-assisted surgery (33). Importantly, however, it has been shown that the learning curve might be steeper in inexperienced learners in skill acquisition in robot surgery (34). This has also been replicated with dedicated microsurgical robot systems. Frieberg et al. showed that anastomosis times were significantly shorter for expert microsurgeons performing manual anastomosis, but that intermediate and novice surgeons performed similarly to the experts when using robot assistance, suggesting that basic microsurgical skills are easier to learn using robot assistance than using manual techniques (35). Importantly, this might make microsurgical treatment modalities available to more surgeons, and thus to a greater number of patients worldwide. Seeing as a considerable amount of training is needed to perform surgery using robot assistance, further technological advances can hopefully enable training in a simulated environment, which could help mitigate the cost of implementation.
A challenge described with the current robotic system is the lack of haptic feedback, or touch sensation (24). This however can be overcome by developing a sense of “see-feel”, as described by Lindenblatt, since the power needed to manipulate the controls of the robot was perceived to match the force needed to use conventional microsurgical instruments (24).
The field of robotics in LVA surgery is young and importantly, the studies that have been done to date have not aimed to prove the superiority of robot-assisted anastomosis, but rather to show that clinical use of robotics is safe and feasible. This is an important aspect of interpreting the results since studies thus far are small and operations have generally been performed by highly experienced microsurgeons. Larger studies including surgeons with varying degrees of experience of both robotic surgery and microsurgery will help determine the place for robot assistance in microsurgery, and whether it can be generally implemented or if it should be reserved for select cases.
Furthermore, the use of robotics in LVA can be seen as an important part of the foundation of robotics in lymphatic supermicrosurgery. Improvements in the LVA using robotic assistance can enable smaller anastomosis and potentially dissemination of the technique to more centers, but the value of the robotic LVA extends beyond the foundational steps, as any advances made in this field could potentially be carried over to more complex procedures.
Overall, the existing robotic systems are an excellent starting point in the relatively new field of robotic microsurgery. Tremor filtration and motion scaling are considered to be the necessary basal functions of a surgical robot, as they address the two most overt limitations of the human hand. Further improvements that might benefit the surgeon include better ergonomics, further increase in precision as well haptic feedback. In addition to larger clinical studies, future research should also be directed towards the cost of implementation to determine at what point the use of a robotic system can be seen as a cost-effective measure, as the expenses associated with starting a robotic microsurgical program might be one of the biggest hurdles for many departments. As technology advances, direct comparisons between the different robotic systems would also be of value.
Conclusions
The use of robotics as an aid in performing supermicrosurgical procedures, such as the LVA, has shown promising results through clinical studies. Though motion scaling and tremor filtration, the techniques can provide better accuracy during the most delicate surgical steps and might help augment the technical capacity of a surgeon, opening up new frontiers in reconstructive microsurgery by overcoming the confines of human dexterity. The learning curve is steep, even for inexperienced learners, and robot assistance could make microsurgery available to more patients. Efforts should be made to increase the availability of the LVA procedure and broader access to robotic assistance might help in this ambition. Further development includes better ergonomics, additional increase in precision as well haptic feedback. Cost optimization and structured training could increase the availability of the technology and will be an important aspect to the spread applicability of robot-assisted microsurgery.
Acknowledgments
Funding: None.
Footnote
Provenance and Peer Review: This article was commissioned by the Guest Editors (Tine Engberg Damsgaard, C. Andrew Salzberg and Jørn Bo Thomsen) for the series “Hot Topics in Breast Reconstruction World Wide” published in Gland Surgery. The article has undergone external peer review.
Reporting Checklist: The authors have completed the Narrative Review reporting checklist. Available at https://gs.amegroups.com/article/view/10.21037/gs-24-22/rc
Peer Review File: Available at https://gs.amegroups.com/article/view/10.21037/gs-24-22/prf
Conflicts of Interest: Both authors have completed the ICMJE uniform disclosure form (available at https://gs.amegroups.com/article/view/10.21037/gs-24-22/coif). The series “Hot Topics in Breast Reconstruction World Wide” was commissioned by the editorial office without any funding or sponsorship. M.M. is on the Medical Advisory Board of Microsure since the summer of 2022 and also a board member of Swedish Microsurgery Board. The authors have no other conflicts of interest to declare.
Ethical Statement: The authors are accountable for all aspects of the work in ensuring that questions related to the accuracy or integrity of any part of the work are appropriately investigated and resolved.
Open Access Statement: This is an Open Access article distributed in accordance with the Creative Commons Attribution-NonCommercial-NoDerivs 4.0 International License (CC BY-NC-ND 4.0), which permits the non-commercial replication and distribution of the article with the strict proviso that no changes or edits are made and the original work is properly cited (including links to both the formal publication through the relevant DOI and the license). See: https://creativecommons.org/licenses/by-nc-nd/4.0/.
References
- Greene AK, Slavin SA, Brorsson H, editors. Lymphedema: Presentation, Diagnosis, and Treatment. Springer; 2015.
- Grada AA, Phillips TJ. Lymphedema: Pathophysiology and clinical manifestations. J Am Acad Dermatol 2017;77:1009-20. [Crossref] [PubMed]
- Senger JB, Kadle RL, Skoracki RJ. Current Concepts in the Management of Primary Lymphedema. Medicina (Kaunas) 2023;59:894. [Crossref] [PubMed]
- Fu MR, Ridner SH, Hu SH, et al. Psychosocial impact of lymphedema: a systematic review of literature from 2004 to 2011. Psychooncology 2013;22:1466-84. [Crossref] [PubMed]
- Damstra RJ, van Steensel MA, Boomsma JH, et al. Erysipelas as a sign of subclinical primary lymphoedema: a prospective quantitative scintigraphic study of 40 patients with unilateral erysipelas of the leg. Br J Dermatol 2008;158:1210-5. [Crossref] [PubMed]
- Soo JK, Bicanic TA, Heenan S, et al. Lymphatic abnormalities demonstrated by lymphoscintigraphy after lower limb cellulitis. Br J Dermatol 2008;158:1350-3. [Crossref] [PubMed]
- Sharma A, Schwartz RA. Stewart-Treves syndrome: pathogenesis and management. J Am Acad Dermatol 2012;67:1342-8. [Crossref] [PubMed]
- Stout NL, Weiss R, Feldman JL, et al. A systematic review of care delivery models and economic analyses in lymphedema: health policy impact (2004-2011). Lymphology 2013;46:27-41. [PubMed]
- Charles R. Elephantiasis Scroti. London: Churchill; 1912.
- Gillies H, Fraser FR. Treatment of lymphoedema by plastic operation: (a preliminary report). Br Med J 1935;1:96-8. [Crossref] [PubMed]
- Yamada Y. The Studies on Lymphatic Venous Anastomosis in Lvmphedema. Nagoya J Med Sci 1969;32:1-21.
- Koshima I, Inagawa K, Urushibara K, et al. Supermicrosurgical lymphaticovenular anastomosis for the treatment of lymphedema in the upper extremities. J Reconstr Microsurg 2000;16:437-42. [Crossref] [PubMed]
- Pons G, Tang JB. Major Changes in Lymphatic Microsurgery and Microvascular Surgery in Past 20 Years. Clin Plast Surg 2020;47:679-83. [Crossref] [PubMed]
- Imai H, Kawase T, Yoshida S, et al. Peripheral T cell profiling reveals downregulated exhaustion marker and increased diversity in lymphedema post-lymphatic venous anastomosis. iScience 2023;26:106822. [Crossref] [PubMed]
- Chang EI, Skoracki RJ, Chang DW. Lymphovenous Anastomosis Bypass Surgery. Semin Plast Surg 2018;32:22-7. [Crossref] [PubMed]
- Peters BS, Armijo PR, Krause C, et al. Review of emerging surgical robotic technology. Surg Endosc 2018;32:1636-55. [Crossref] [PubMed]
- Katz RD, Rosson GD, Taylor JA, et al. Robotics in microsurgery: use of a surgical robot to perform a free flap in a pig. Microsurgery 2005;25:566-9. [Crossref] [PubMed]
- van der Hulst R, Sawor J, Bouvy N. Microvascular anastomosis: is there a role for robotic surgery? J Plast Reconstr Aesthet Surg 2007;60:101-2. [Crossref] [PubMed]
- Karamanoukian RL, Finley DS, Evans GR, et al. Feasibility of robotic-assisted microvascular anastomoses in plastic surgery. J Reconstr Microsurg 2006;22:429-31. [Crossref] [PubMed]
- Taleb C, Nectoux E, Liverneaux P. Limb replantation with two robots: a feasibility study in a pig model. Microsurgery 2009;29:232-5. [Crossref] [PubMed]
- Katz RD, Taylor JA, Rosson GD, et al. Robotics in plastic and reconstructive surgery: use of a telemanipulator slave robot to perform microvascular anastomoses. J Reconstr Microsurg 2006;22:53-7. [Crossref] [PubMed]
- van Mulken TJM, Schols RM, Scharmga AMJ, et al. First-in-human robotic supermicrosurgery using a dedicated microsurgical robot for treating breast cancer-related lymphedema: a randomized pilot trial. Nat Commun 2020;11:757. [Crossref] [PubMed]
- Hong JP, Pak CJ, Suh HP. Supermicrosurgery in Lower Extremity Reconstruction. Clin Plast Surg 2021;48:299-306. [Crossref] [PubMed]
- Lindenblatt N, Grünherz L, Wang A, et al. Early Experience Using a New Robotic Microsurgical System for Lymphatic Surgery. Plast Reconstr Surg Glob Open 2022;10:e4013. [Crossref] [PubMed]
- Barbon C, Grünherz L, Uyulmaz S, et al. Exploring the learning curve of a new robotic microsurgical system for microsurgery. JPRAS Open 2022;34:126-33. [Crossref] [PubMed]
- van Mulken TJM, Wolfs JAGN, Qiu SS, et al. One-Year Outcomes of the First Human Trial on Robot-Assisted Lymphaticovenous Anastomosis for Breast Cancer-Related Lymphedema. Plast Reconstr Surg 2022;149:151-61. [Crossref] [PubMed]
- Weinzierl A, Barbon C, Gousopoulos E, et al. Benefits of robotic-assisted lymphatic microsurgery in deep anatomical planes. JPRAS Open 2023;37:145-54. [Crossref] [PubMed]
- Marshall J, Walsh EG. Physiological tremor. J Neurol Neurosurg Psychiatry 1956;19:260-7. [Crossref] [PubMed]
- Gundlapalli VS, Ogunleye AA, Scott K, et al. Robotic-assisted deep inferior epigastric artery perforator flap abdominal harvest for breast reconstruction: A case report. Microsurgery 2018;38:702-5. [Crossref] [PubMed]
- Hughes T, Rai B, Madaan S, et al. The Availability, Cost, Limitations, Learning Curve and Future of Robotic Systems in Urology and Prostate Cancer Surgery. J Clin Med 2023;12:2268. [Crossref] [PubMed]
- Lam K, Clarke J, Purkayastha S, et al. Uptake and accessibility of surgical robotics in England. Int J Med Robot 2021;17:1-7. [Crossref] [PubMed]
- van Mulken TJM, Schols RM, et al. Robotic (super) microsurgery: Feasibility of a new master-slave platform in an in vivo animal model and future directions. J Surg Oncol 2018;118:826-31. [Crossref] [PubMed]
- Alrasheed T, Liu J, Hanasono MM, et al. Robotic microsurgery: validating an assessment tool and plotting the learning curve. Plast Reconstr Surg 2014;134:794-803. [Crossref] [PubMed]
- Kaul S, Shah NL, Menon M. Learning curve using robotic surgery. Curr Urol Rep 2006;7:125-9. [Crossref] [PubMed]
- Frieberg H, Nilsson A, Önefäldt D, et al. Robot-Assisted Microsurgery – What Does the Learning Curve Look Like? Journal of Plastic and Reconstructive Surgery 2023. doi:
10.1016/j.bjps.2023.10.019 .10.1016/j.bjps.2023.10.019