Indocyanine green-enhanced fluorescence for assessing parathyroid perfusion during thyroidectomy
Introduction
Recently, indocyanine green (ICG)-enhanced fluorescence has been used to improve visualization and to provide detailed anatomical information during different surgical procedures, especially laparoscopic surgery (1-8). Fluorescent optical guidance minimizes inadvertent tissue injury while enhancing procedural efficiency (9).
Indocyanine green is a water-soluble anionic amphiphilic tricarbocyanine dye with a molecular weight of 774.96 Da (1-9). This dye can be injected into the human bloodstream with practically no adverse effects (10-19) and becomes fluorescent upon excitation by a laser beam or by light with a wavelength in the near infra-red (NIR) spectrum (approximately 820 nm). This dye was first approved for clinical use in 1956 and is now the only clinically approved NIR fluorescent dye (9). Fluorescence is detected by specialized scopes and cameras and then transmitted to a standard monitor to enable identification of anatomical structures where the dye is present (i.e., biliary ducts, vessels, lymph nodes, etc.). Intravenous injection of ICG during a surgical procedure enables real-time assessment of visceral blood supply and evaluation of bowel perfusion (1-9). Injected ICG binds tightly to lipoproteins and is rapidly excreted into the bile (1-8).
ICG-enhanced fluorescence imaging in surgery
Using ICG to enhance fluorescence imaging enables real-time assessment and direct imaging of tissue perfusion and vascularization (1-8). The use of ICG-enhanced fluorescence imaging in general surgery has also increased because of its well-established safety profile (10-19). The many applications of ICG-enhanced fluorescence imaging in guided surgical procedures include intraoperative angiography, laparoscopic cholecystectomy, extrahepatic cholangiography, colorectal resection, living-donor nephrectomy, laparoscopic kidney autotransplantation, lymph node mapping and dissection, and various other procedures (9,20,21). Indocyanine green-enhanced fluorescence imaging is now well established as simple, inexpensive, and effective (1-8) and is expected to become a standard technique for many diagnostic and oncological purposes in the near future. However, further large scale studies are needed to clarify its specific advantages and disadvantages in large populations.
Cholecystectomy
For a safe cholecystectomy procedure, confident identification of the extrahepatic biliary anatomy is mandatory. The use of fluorescein and ultraviolet light improves visualization of the biliary topography during laparoscopic cholecystectomy (22-26). Within 4 to 5 min after application of fluorescein, the bile ducts emit a green fluorescence upon exposure to ultraviolet A light from an LED light source. The fluorescence enables clear differentiation between the biliary system and surrounding tissues (22-26). The extrahepatic biliary anatomy can be identified faster by fluorescence technique than by conventional white light. Tissues remain fluorescent for 42 to 77 min after application of fluorescein, which is a sufficient duration to complete most laparoscopic cholecystectomy procedures (22-26). Compared to white light, the fluorescence technique allows better and earlier real-time visualization of the biliary anatomy. Fluorescence technique provides an additional tool for improving the safety of laparoscopic cholecystectomy. Generally, fluorescence technique can clearly distinguish the biliary-vascular anatomy, and no intraoperative or injection-related adverse effects have been reported (22-26). The invaluable information provided by this imaging system improves the safety of cholecystectomy and is useful for planning surgery. Additionally, no case series studies of this procedure have reported bile duct injuries (22-26).
Colorectal surgery
Anastomotic leakage after colorectal surgery is a severe complication. A possible cause of anastomotic leakage is insufficient vascular supply. An ICG-enhanced fluorescent angiography provides useful intraoperative information about vascular perfusion during colorectal surgery. Angiographic data may indicate the need to change the resection site or the need to perform anastomosis, which may then affect the anastomotic leakage rate (27). An interesting study by Boni et al. [2016] evaluated the feasibility of using ICG-enhanced fluorescence for intraoperative assessment of vascular anastomotic perfusion in colorectal surgery (27). Anatomical structures were visualized by a high definition stereoscopic camera connected to a 30° 10 mm scope equipped with a specific lens and a light source emitting both visible light and NIR light (KARL STORZ GmbH & Co. KG, Tuttlingen, Germany). After injection of ICG, the system projected high-resolution NIR images of blood flow in vessels and organs in real time, which highlighted sites of biliary excretion (27). All anastomosis sites and resection margins in colorectal surgery were investigated by intraoperative fluorescence angiography to assess colonic perfusion before and after anastomosis and in both right and left colectomies (27). The study enrolled 107 patients undergoing colorectal laparoscopic resection, including 40 right colectomies, 10 splenic flexure segmental resections, 35 left colectomies, and 22 anterior resections. In 90% of cases, the indication for surgery was cancer, and high ligation of vessels was performed (27). Based on the fluorescence intensity, the surgical team concluded that perfusion of the distal part of the proximal bowel to be anastomosed was insufficient in 4/107 patients (two anterior, one sigmoid and one segmental splenic flexure resections for cancer).
Thus, further proximal “re-resection” was performed up to a “fluorescent” portion (27). None of these patients had a clinical leak. The overall morbidity rate was 30%; one patient undergoing right colectomy had anastomotic leakage that was apparently unrelated to ischemia; no clinical signs of anastomotic leakage were observed in the colorectal resections, including the low anterior resections (27).
Sleeve gastrectomy
A study by Frattini et al. presented their preliminary experience using indocyanine green fluorescence (IGF) imaging in laparoscopic sleeve gastrectomy (28). After dissection of the greater curve sleeve, gastrectomy was performed using a linear articulated stapler. After resection of the stomach, IGF solution was prepared and injected into a periferic vein. The laparoscopic system used in all procedures included a high-definition camera connected to a laparoscope equipped with a filter specifically optimized for detection of NIR fluorescence (28). A methylene blue test was routinely performed after detection of NIR fluorescence, and a routine gastrografin upper gastrointestinal study was performed on postoperative day 2. A retrospective analysis of IGF imaging results for 15 patients who had received laparoscopic sleeve gastrectomy showed that perfusion was regular and homogeneous along the entire gastric sleeve, including the esophagogastric junction (28). In contrast, IGF imaging revealed a devascularized appearance in excised specimens, which was consistent with expectations. Intraoperative methylene blue test was negative in all cases. No leaks occurred during contrast swallow. Additionally, no leaks or other complications occurred postoperatively (minimum follow up, 2 months). This report of a preliminary experience using IGF indicated that the results were consistent with those of intraoperative methylene blue and postoperative contrast swallow tests. Moreover, it showed that IGF can help to identify the exact pathogenesis of a gastric leak (28).
Sentinel lymph node (SLN) biopsy
Reliable methods for clinical detection of the SLN are in great demand to avoid unnecessary resection (29-34). Indocyanine green is commonly used in SLN biopsies for different oncological procedures. The metastatic status of regional lymph nodes is the most relevant prognostic factor in breast cancer, melanoma, and other solid organ tumors with a lymphatic spread (29-34). The current gold standard for detection and targeted excision of the SLN is preoperative lymphoscintigraphy with technetium Tc 99m (29). Because of the worldwide shortage of technetium Tc 99m, however, physicians are evaluating the use of nonradioactive dyes for SLN labeling (29). Several retrospective studies have indicated that ICG is a feasible alternative to technetium Tc 99m (29). For example, Stoffels et al. [2015] compared intraoperative NIR fluorescence sentinel lymph node excision (SLNE), which is well established as effective in malignant melanoma, and standard technetium Tc 99m-guided SLNE in terms of feasibility and clinical benefit (29). A prospective clinical trial at the Skin Cancer Center, University Hospital Essen analyzed 80 patients scheduled to undergo SLNE for treatment of malignant melanoma on the trunk or extremities (upper and lower) from January 1, 2013 to June 27, 2014 (29). The authors reported a high concordance of preoperative and intraoperative SLN detection rates. During the study period, 80 patients received surgery with an additional intraoperative application of an NIR fluorescent dye. In these 80 surgical procedures, 147 SLNs were excised (29). In all cases, a technetium Tc 99m-marked SLN was detected before surgery (29). However, the SLN could be visualized intraoperatively by ICG before skin incision in only 17 of 80 (21%) of the patients. The NIR fluorescence technique successfully identified the SLN in the operative site after skin incision and initial tissue preparation in 141 of 147 (96%) cases. The authors concluded that, when the lymph node basin cannot be predicted correctly (e.g., in cutaneous melanoma on the trunk), ICG is more effective for SLNE than for SLN detection when using standard technique guided by technetium Tc 99m (29). Therefore, radiocolloid, followed if possible by single-photon emission computed tomography, remains the gold standard for SLNE (29).
Another study evaluated the feasibility of fluorescence detection of SLNs with ICG for lymphatic mapping and SLN biopsy for axillary staging in breast cancer (30-32). Thirty women with breast cancer received a periareolar injection of ICG for fluorescence detection of SLN with an NIR camera (30-32). Another twenty received (99m)Tc-labelled sulphur radiocolloid for SLN scintigraphy (30-32). All patients underwent axillary lymph node dissection. The detection rates and sensitivities of both methods were compared at the study endpoints (30-32). Visualization of lymphatic vessels by fluorescence detection depended on the ICG dose. The ICG imaging identified SLNs in 29 of 30 women (detection rate, 97%). Additionally, 19 of 21 patients had metastatic SLN involvement (sensitivity, 90%) with false-negative results in two. In the 20 patients examined by both methods, ICG fluorescence and radiocolloid identified SLNs in 20 and 17 patients, respectively. The ICG fluorescence and radiocolloid identified metastatic lymph nodes in 12 and ten of 13 patients (92% and 77% sensitivity, respectively). False-negative rates were 8% and 23%, respectively. The author concluded that, since ICG fluorescence can be used for both transcutaneous imaging of lymphatic vessels and SLN detection, it combines the advantages of radioisotope and blue dye methods (30-32). Indocyanine green fluorescence imaging has also been evaluated for use in gastric cancer (33). In a clinical exploration/feasibility study of a novel ICG fluorescence imaging system, an SN biopsy was performed using ICG dye in three patients scheduled to undergo surgery for gastric cancer. The system used to detect ICG fluorescence comprised a charge-coupled device (CCD) camera with a cut filter as the detector and light emitting diodes (LEDs) as the light source (34). The nodes were also examined simultaneously by an infrared (IR) imaging videoscope. The fluorescence imaging system detected movement of the ICG dye immediately after injection and enabled clear visualization of the lymphatic vessels draining from the primary gastric tumor toward the lymph nodes. Some lymph vessels and nodes were not clearly distinguishable by either ICG imaging or IR imaging (34). As in radio-guided method, the ICG fluorescence system also enabled visualization of the lymph node when ICG was injected the day before surgery. The proposed ICG fluorescence imaging system has promising applications for detecting SNs in gastric cancer surgery and may also prove useful for laparoscopic surgery (34).
A lateral pelvic lymph node dissection (LPLD) for lower rectal cancer may be beneficial for a limited number of patients (35). Performing SN navigation surgery in lower rectal cancer may avoid unnecessary LPLD. An earlier study investigated the feasibility of using an NIR camera system and ICG to visualize the lateral region of an SN biopsy sample (Photodynamic Eye, PDE) (35). Lateral region SNs were investigated in 25 patients with lower rectal cancer by injecting ICG near the tumor and then observing the lateral pelvic region under PDE. Under PDE, the lymph nodes and lymph vessels exposed to ICG appeared as bright fluorescent spots and streams in the fluorescence image. Both tumor-negative and tumor-positive SNs also appeared as bright fluorescent spots (35). Lateral SNs were detected in 6 of 6 T1 and T2 diseases and in 17 of 19 T3 diseases. The lateral SNs were successfully identified in 23 (92%) of the 25 patients. The mean number of lateral SNs per patients was 2.1. Of the 23 patients, 6 patients received LPLD. Dissected lateral non-SNs were negative in all three patients with tumor-negative SNs (35). The authors successfully detected lateral SNs not only in T1 and T2 disease, but also in T3 disease. Although this was only a preliminary study, the successful use of ICG fluorescence imaging to detect lateral SNs in patients with lower rectal cancer shows that ICG fluorescence imaging has promising applications for assessing whether patients require LPLD (35).
Applications of ICG-enhanced fluorescence imaging in thyroid surgery
Parathyroid function after thyroid surgery
Postoperative hypocalcemia is a common complication of thyroidectomy performed by either conventional or endoscopic methods (35-41). Decreased serum calcium, secondary to hypoparathyroidism, may present clinically with muscle cramps, perioral and peripheral paresthesias, carpopedal spasm or tetany, and/or confusion. Symptomatic patients often require extended hospitalization after thyroid surgery, which increases healthcare costs (35-41). Depending on the extent of parathyroid gland damage, hypocalcemia may be transient and resolve within several few months or may be permanent. Permanent hypocalcemia requires lifelong oral calcium and vitamin D supplementation. Although ambulatory thyroid surgery enables early discharge of patients, postoperative hypocalcemia is a particularly important consideration (35-41). In fact, some surgeons advocate indiscriminate postoperative calcium supplementation. However, this approach has been contested. On result of the increased interest in outpatient and short-stay thyroid surgery is the improved ability of surgeons to identify patients at risk for hypocalcemia (35-41). Therefore, accurate and standardized data for thyroidectomy outcomes are essential. Unfortunately, although hypocalcemia is well documented in the thyroid surgery literature, previous studies have had notable limitations. Perhaps the most notable limitation is the wide range of reported incidence rates for hypocalcemia after thyroid surgery (0.3–66.2%). A likely explanation for this variation is that different reports assess different thyroid surgery procedures. For example, some studies analyze not only patients who have received total thyroidectomy, but also patients who have received less invasive procedures such as thyroid lobectomy, which has a relatively low hypocalcemia risk. Such reports can underestimate the incidence of hypocalcemia and are subject to misinterpretation (35-41).
The current literature indicate that the incidence of hypocalcemia may be affected by several risk factors. For example, although the exact mechanism is unclear, the incidence of hypocalcemia is reportedly highest in the most invasive thyroidectomy procedures. Other potentially predictive variables of postoperative hypocalcemia, such as iatrogenic parathyroidectomy, have shown no effects on patient outcomes. Although some experts posit that the skill and experience of the surgeon affect the occurrence of postthyroidectomy hypocalcemia, one study found that the complications of surgical procedures performed by trainees were comparable to those of procedures performed by consultant surgeons (35-41).
Insufficient blood perfusion and insufficient venous drainage have been highlighted as potential risk factors for postoperative parathyroid malfunction (9,35-41). Although identifying the causes of postoperative hypoparathyroidism is difficult, if not impossible, inadequate parathyroid gland perfusion is a very common cause (9,35-41). Intraoperative assessment of parathyroid gland perfusion is usually subjectively estimated by the surgeon according to the color of the gland and visible bleeding at the edge of the gland (9,35-41).
Use of ICG-enhanced fluorescence for evaluating parathyroid gland preservation
Different techniques for assessing optimal parathyroid gland perfusion have been proposed (9). First, all procedures involving the thyroid gland require accurate and precise identification of the parathyroid glands (9,35-41). Identifying the parathyroid glands during thyroid surgery may prevent their inadvertent surgical removal and thus provide a better postoperative quality of life. Nevertheless, the “technique” most commonly used to evaluate parathyroid gland tissue perfusion is the judgement of the surgeon based on intraoperative clinical findings and based on visual observations such as color, bleeding edges, pulsation and temperature. Both laser Doppler flowmetry and oxygen spectroscopy have been used for this purpose but have several methodologic limitations. Another approach is intraoperative measurements of intact parathyroid hormone.
Fluorescence angiography is performed by using dedicated cameras and scopes for direct visualization of the fluorescence emitted by specific fluorophores such as ICG under NIR light (9,35-41). During surgery, this technique also has potential use in real-time angiography for evaluating parathyroid gland perfusion (9,35-41).
Lessons from experimental studies
A study performed at Seoul National University Hospital used a canine model to evaluate the use of ICG for NIR fluorescent imaging of the parathyroid gland (9). This study is definitely the first preclinical study in which ICG and NIR fluorescent imaging were used to visualize parathyroid glands. The authors compared the fluorescence intensity in response to varying ICG doses. Their data analyses revealed the optimal concentration of ICG for visualizing the parathyroid gland in dogs, and the authors were able to obtain a reproducible time to peak intensity (9). In detail, three dogs were used for the study (9). After general anesthesia and surgical dissection, each dog received a series of intravenous ICG doses ranging from 12.5 to 100 µg/kg ICG. In detail, to obtain NIR imaging 25 mg of ICG (Indocyanine Green Injection; Dongindang Pharmaceutical Co., Siheung-si, Korea) was dissolved in 10 mL sterile water. Individual doses of 12.50, 18.75, 25.00, 50.00, and 100.00 µg/kg were administered intravenously (9). The external parathyroid gland was illuminated by a 785 nm, 500 mW diode laser (9). After each administration of an ICG dose, tissue fluorescence was assessed (9). Real-time fluorescent images were obtained using a 1394 ORCA-ER CCD camera (Hamamatsu Photonics, Hamamatsu, Shizuoka, Japan) and a wide-zoom lens (Nikon, Nikon Vision Co., Tokyo, Japan) equipped with an 835/45 nm band-pass filter (9). After the imaging procedure, the dogs were euthanized. External parathyroid glands, thyroid glands, and recurrent laryngeal nerves (RLNs) were resected and fixed in 10% formaldehyde. Sections were stained using hematoxylin and eosin (H&E) and examined by a pathologist (9). The excitation light source used to illuminate the operating field was an NIR laser (λ=785 nm). Intravascular ICG fluorescence (λ=835/45 nm) was recorded using a CCD with an optical filter for blocking ambient and laser light (9). Fluorescent imaging was assessed after each injected ICG dose. The intensity curves of the NIR fluorescent images of the parathyroid glands showed similar peaks and plateaus of fluorescence, regardless of ICG concentration. The time to peak fluorescent intensity was 50.2±2.0 s after injection of ICG. Considering background fluorescent intensity, the estimated optimal dose of ICG was 18.75 µg/kg. At 106.7±5.8 s, the fluorescence of the parathyroid glands was substantially reduced but was still distinguishable (9). Fluorescent intensity correlated positively with increasing ICG doses up to 25 µg/kg. The authors concluded that ICG NIR fluorescent imaging was useful for detecting the parathyroid gland in dogs and has potential applications as an aid for thyroidectomies performed by endocrine surgeons (9). However, the authors of this preclinical study cautioned that further clinical trials are warranted (9).
From experimental studies to human studies
A pilot study will be performed to assess the use of ICG enhanced fluorescence for comparing parathyroid gland perfusion before and after thyroidectomy. Further objectives of the study will include evaluating whether this technology affects the success of the surgeon in preserving and re-implanting thyroid glands and whether it subsequently decreases the rate of parathyroid malfunction after thyroid surgery. The participants will be patients undergoing total thyroidectomy for different thyroid diseases. Recruitment of participants for this pilot study will begin in September 2016. The recruitment criteria will be age 18 years or older and referral for thyroidectomy. The exclusion criteria will be pregnancy or intention to become pregnant in the future as well as any history of adverse reaction or known allergy to ICG, iodine or iodine dyes. Participants will be asked to review and sign informed consent forms before beginning the study. Data for both endoscopic and conventional open thyroidectomy will be collected and entered in a specifically designated database. The following parameters will be recorded for the baseline assessment: demographic data (age, gender, race), BMI, ASA class, preoperative diagnosis, history of preoperative chemo- or radiotherapy, tobacco or alcohol use, complete medical history. Surgical techniques will be performed according to the standard practice of the surgeon and the diagnosis of the patient. Conversion to open surgery will not be an exclusion criterion. A detailed description of the surgical technique will be essential, including operative time, parathyroid gland mobilization, type of parathyroid dissection, type of energy based device (EBD), number of coagulations/EBD applications used for parathyroid gland division, and number of parathyroid glands visualized, re-implantated and irrigated.
The operative protocol will be as follows: upon identification of a thyroid gland (per side), a bolus of ICG will be injected IV in a peripheral vein of the left or right arm. The amount of ICG to be injected will be precisely calculated preoperatively according to the weight of the patient at a fixed dose of 0.2 mg/kg. When the first parathyroid gland is identified, all lights in the operating theatre will be turned off, and the operating surgeon will use the fluorescence system to estimate parathyroid gland perfusion (PGP 1). Time (seconds) between ICG injection and first appearance of fluorescence will be recorded to provide an indirect marker of vascular failure. The operating surgeon will subjectively evaluate the perfusion of the previously identified site of parathyroid gland and rate the fluorescence as “good”, “blurry” or “absent” in PGP2 in comparison to PGP1. This phase of the procedure will be recorded in HD recorded and analyzed by the coordinating center by using software specifically designed to provide an objective measure of fluorescence intensity http://www.icy.bioimageanalysis.org. Finally, gland preservation or re-implantation will be performed using the technique preferred by the surgeon.
Available enhanced fluorescence systems
Spies, Karl Storz (KARL STORZ GmbH & Co. KG, Tuttlingen, Germany)
An image can be generated by a commercially available high-end full high definition camera system (IMAGE 1 SPIES, KARL STORZ) connected to a laparoscope with 30° or 0° field of direction and 10 mm diameter. The laparascope is equipped with a specific filter for optimal detection of NIR fluorescence and white light without manual switching. The IMAGE1 SPIES combines an HD camera and a laparoscope in a single system for all applications. Because the IMAGE1 SPIES camera platform is modular, it can integrate various technologies (e.g., rigid, flexible and 3D endoscopy) in a single system, which makes it highly adaptable to individual customer needs. The camera platform can also combine NIR with ICG for fluorescence imaging and can integrate operating microscopes with VITOM® exoscopes. Its xenon light source (D-LIGHT P SCB, KARL STORZ) provides both visible and NIR excitation light. The surgeon can use a footswitch to change from standard light to NIR. An image enhancement system is included to improve visualization under both visible light in standard mode and under NIR light (IMAGE 1 SPIES system, KARL STORZ GmbH & Co. KG, Tuttlingen, Germany), and the visualization modalities can be easily adjusted according to the needs and preferences of the surgeon.
Spy System—Novadaq (Novadaq, 11091 Corsia Trieste Way, Unit 201 Bonita Springs, Florida, USA)
The SPY Elite (Figure 1) provides surgeons with intraoperative visualization of microvascular blood flow and tissue perfusion when performing gastrointestinal surgery, cardiothoracic surgery, or open procedures such as reconstruction of the breast or thyroid. The innovative SPY-Q software used in the SPY Elite provides surgeons with a range of on-screen tools for objectively analyzing blood flow. This objective data can complement the direct clinical observations of the surgeon by providing a comprehensive view of perfusion. Contour mapping, for example, allows surgeons to visualize different levels of blood flow in the tissue according to contour thresholds. Because the SPY Elite is small and highly portable, it can be used for fluorescence imaging throughout the OR. The operating room staff can manage the setup so that the surgeon can focus on controlling the articulating arm and camera head. In addition to providing intraoperative visualization of blood flow, the high-quality images captured and streamed to the SPY Elite’s high definition displays are archived for further detailed analysis. Additionally, unlike other imaging devices, the SPY Elite does not emit ionizing radiation and does not require use of radiation protective gear. After the imaging head is positioned over the patient, the system emits an NIR excitation light and illuminates the area of interest. When the patient is injected with ICG fluorescence imaging agent, the ICG binds to the plasma in blood and travels through the bloodstream to the area of interest. Indocyanine green is a sterile, water soluble, tricarbocyanine dye with a peak spectral absorption at 800–810 nm in blood plasma or blood. The fluorescence response induced by exposure of ICG to NIR excitation light emitted by the SPY imaging device is then captured by the NIR camera in the imaging head. The resulting image signal is processed and simultaneously recorded in computer memory and displayed on the video monitors in real time. After injection, ICG rapidly binds to plasma proteins, primarily lipoproteins with a lesser and variable binding to albumin (2–30% of total). Simultaneous arterial and venous blood estimations have shown negligible renal, peripheral, lung, or cerebrospinal uptake of the dye. Hepatic parenchymal cells take up almost all ICG from plasma and secrete it into the bile. Enterohepatic recirculation of ICG is negligible, and ICG has a normal biological half-life of 2.5–3.0 min. The SPY System includes a SPY-Q software package application for viewing, manipulating, measuring and comparing medical image sequences produced at single or multiple time-points by the Fluorescence Imaging Device. Entire image time-sequences are processed by various analytical algorithms that provide visual or numerical results complementing the clinical perspective of the surgeon. The contour lines in the image represent lines of isofluorescence, and all points on a contour have equal fluorescence.
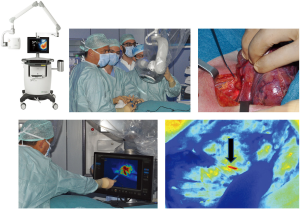
Striker endoscopic near-infrared visualization (ENV, Stryker Endoscopy, San Jose, CA, USA)
The Striker ENV system provides enhanced real-time visualization of anatomy during minimally invasive surgery. When ENV mode is activated, the system uses fluorescent light to visualize blood flow, tissue perfusion, and biliary ducts. The Striker ENV system is used with a fluorescent ICG dye. The system consists of an ENV light source, light cable, camera control unit, 1488 camera head, coupler, 0° and 30° 10 mm laparoscopes, and surgical display unit. The system illuminates the surgical site by generating light within visible and infrared spectra. After image signals are transmitted from the laparoscope to the camera control unit for processing, the final image is displayed on the monitor. A button on the camera head can be used to toggle between visible light and NIR light.
Firefly fluorescence imagining for robots (da Vinci® Si™ Surgical System, Intuitive Surgical, Inc., Sunnyvale, California, USA)
The new Firefly technology integrated in the INTEGRIS da Vinci® Si™ Surgical System enables the use of NIR technology for real-time, image-guided identification of key anatomical landmarks.
The ICG is introduced through a peripheral IV line and then binds to plasma proteins in blood. The ICG dye, which has a “firefly green” appearance under a special fluoroscopic robotic camera, can differentiate between cancerous and healthy tissues and can indicate the blood supply to the tumor. Therefore, the system improves the precision of tumorectomy. That is, it can potentially lower the risk of removing healthy tissue of an organ and the risk of incomplete removal of cancer. The operator can quickly switch between Firefly imaging and visualization under normal white light. Compared to the naked eye, fluorescence imaging provides surgeons with a better view of the anatomy and further enhances the already unmatched vision, precision and control of minimally invasive da Vinci® surgery.
Conclusions
Indocyanine green-enhanced fluorescence imaging merits careful evaluation for use in endocrine surgery, especially in thyroid surgery because thyroid surgery has a high incidence of hypocalcemia, and hypocalcemia is usually the first complication after surgery. This technique is inexpensive, safe, noninvasive and non-radiological.
Fluorescence imaging enables surgeons to capture, review and archive high-quality fluorescence images of circulation, including lymphatics and blood vessels, and related tissue perfusion during thyroid surgical procedures.
However, further large randomized prospective trials are needed to validate this new technique.
Primary endpoints of future studies should include evaluation of the efficacy of ICG-enhanced fluorescence for estimating parathyroid gland perfusion before wound closure and for identifying changes in the previously identified gland at the end of surgery.
Secondary endpoints of future studies should include identification of potential technique-related failures or complications such inability to record fluorescence, allergic or other adverse reaction to ICG injection, overall cost of the technique, and the incidence of postoperative hypocalcemia.
By assisting surgeons in making critical decisions in the operating room, this technology can reduce rates of postoperative complications and decrease healthcare costs.
Acknowledgements
None.
Footnote
Conflicts of Interest: The authors have no conflicts of interest to declare.
References
- Adams KE, Ke S, Kwon S, et al. Comparison of visible and near-infrared wavelength-excitable fluorescent dyes for molecular imaging of cancer. J Biomed Opt 2007;12:024017. [Crossref] [PubMed]
- Sevick-Muraca EM, Rasmussen JC. Molecular imaging with optics: primer and case for near-infrared fluorescence techniques in personalized medicine. J Biomed Opt 2008;13:041303. [Crossref] [PubMed]
- Hwang K, Houston JP, Rasmussen JC, et al. Improved excitation light rejection enhances small-animal fluorescent optical imaging. Mol Imaging 2005;4:194-204. [PubMed]
- Houston JP, Thompson AB, Gurfinkel M, et al. Sensitivity and depth penetration of continuous wave versus frequency-domain photon migration near-infrared fluorescence contrast-enhanced imaging. Photochem Photobiol 2003;77:420-30. [Crossref] [PubMed]
- Reynolds JS, Troy TL, Mayer RH, et al. Imaging of spontaneous canine mammary tumors using fluorescent contrast agents. Photochem Photobiol 1999;70:87-94. [Crossref] [PubMed]
- Gurfinkel M, Thompson AB, Ralston W, et al. Pharmacokinetics of ICG and HPPH-car for the detection of normal and tumor tissue using fluorescence, near-infrared reflectance imaging: a case study. Photochem Photobiol 2000;72:94-102. [Crossref] [PubMed]
- Alander JT, Kaartinen I, Laakso A, et al. A review of indocyanine green fluorescent imaging in surgery. Int J Biomed Imaging 2012;2012:940585.
- Luo S, Zhang E, Su Y, et al. A review of NIR dyes in cancer targeting and imaging. Biomaterials 2011;32:7127-38. [Crossref] [PubMed]
- Suh YJ, Choi JY, Chai YJ, et al. Indocyanine green as a near-infrared fluorescent agent for identifying parathyroid glands during thyroid surgery in dogs. Surg Endosc 2015;29:2811-7. [Crossref] [PubMed]
- Hope-Ross M, Yannuzzi LA, Gragoudas ES, et al. Adverse reactions due to indocyanine green. Ophthalmology 1994;101:529-33. [Crossref] [PubMed]
- Obana A, Miki T, Hayashi K, et al. Survey of complications of indocyanine green angiography in Japan. Am J Ophthalmol 1994;118:749-53. [Crossref] [PubMed]
- Albo D, Wayne JD, Hunt KK, et al. Anaphylactic reactions to isosulfan blue dye during sentinel lymph node biopsy for breast cancer. Am J Surg 2001;182:393-8. [Crossref] [PubMed]
- Speich R, Saesseli B, Hoffmann U, et al. Anaphylactoid reactions after indocyanine-green administration. Ann Intern Med 1988;109:345-6. [Crossref] [PubMed]
- Michie DD, Wombolt DG, Carretta RF, et al. Adverse reactions associated with the administration of a tricarbocyanine dye (Cardio-Green) to uremic patients. J Allergy Clin Immunol 1971;48:235-9. [Crossref] [PubMed]
- Bonte CA, Ceuppens J, Leys AM. Hypotensive shock as a complication of infracyanine green injection. Retina 1998;18:476-7. [Crossref] [PubMed]
- Garski TR, Staller BJ, Hepner G, et al. Adverse reactions after administration of indocyanine green. JAMA 1978;240:635. [Crossref] [PubMed]
- Nanikawa R, Hayashi T, Hayashi K. A case of fatal shock induced by indocyanine green (ICG) test. Jap J Leg Medicine 1978;32:209-14.
- Olsen TW, Lim JI, Capone A Jr, et al. Anaphylactic shock following indocyanine green angiography. Arch Ophthalmol 1996;114:97. [Crossref] [PubMed]
- Benya R, Quintana J, Brundage B. Adverse reactions to indocyanine green: a case report and a review of the literature. Cathet Cardiovasc Diagn 1989;17:231-3. [Crossref] [PubMed]
- Boni L, David G, Mangano A, et al. Clinical applications of indocyanine green (ICG) enhanced fluorescence in laparoscopic surgery. Surg Endosc 2015;29:2046-55. [Crossref] [PubMed]
- Reuthebuch O, Häussler A, Genoni M, et al. Novadaq SPY: intraoperative quality assessment in off-pump coronary artery bypass grafting. Chest 2004;125:418-24. [Crossref] [PubMed]
- Liu YY, Kong SH, Diana M, et al. Near-infrared cholecysto-cholangiography with indocyanine green may secure cholecystectomy in difficult clinical situations: proof of the concept in a porcine model. Surg Endosc 2016;30:4115-23. [Crossref] [PubMed]
- Ishizawa T, Bandai Y, Kokudo N. Fluorescent cholangiography using indocyanine green for laparoscopic cholecystectomy: an initial experience. Arch Surg 2009;144:381-2. [Crossref] [PubMed]
- Mohsen AA, Elbasiouny MS, Fawzy YS. Fluorescence-guided laparoscopic cholecystectomy: a new technique for visualization of biliary system by using fluorescein. Surg Innov 2013;20:105-8. [Crossref] [PubMed]
- Daskalaki D, Fernandes E, Wang X, et al. Indocyanine green (ICG) fluorescent cholangiography during robotic cholecystectomy: results of 184 consecutive cases in a single institution. Surg Innov 2014;21:615-21. [Crossref] [PubMed]
- Spinoglio G, Priora F, Bianchi PP, et al. Real-time near-infrared (NIR) fluorescent cholangiography in single-site robotic cholecystectomy (SSRC): a single-institutional prospective study. Surg Endosc 2013;27:2156-62. [Crossref] [PubMed]
- Boni L, David G, Dionigi G, et al. Indocyanine green-enhanced fluorescence to assess bowel perfusion during laparoscopic colorectal resection. Surg Endosc 2016;30:2736-42. [Crossref] [PubMed]
- Frattini F, Lavazza M, Mangano A, et al. Indocyanine green-enhanced fluorescence in laparoscopic sleeve gastrectomy. Obes Surg 2015;25:949-50. [Crossref] [PubMed]
- Stoffels I, Dissemond J, Pöppel T, et al. Intraoperative Fluorescence Imaging for Sentinel Lymph Node Detection: Prospective Clinical Trial to Compare the Usefulness of Indocyanine Green vs Technetium Tc 99m for Identification of Sentinel Lymph Nodes. JAMA Surg 2015;150:617-23. [Crossref] [PubMed]
- Murawa D, Hirche C, Dresel S, et al. Sentinel lymph node biopsy in breast cancer guided by indocyanine green fluorescence. Br J Surg 2009;96:1289-94. [Crossref] [PubMed]
- Sevick-Muraca EM, Sharma R, Rasmussen JC, et al. Imaging of lymph flow in breast cancer patients after microdose administration of a near-infrared fluorophore: feasibility study. Radiology 2008;246:734-41. [Crossref] [PubMed]
- Troyan SL, Kianzad V, Gibbs-Strauss SL, et al. The FLARE intraoperative near-infrared fluorescence imaging system: a first-in-human clinical trial in breast cancer sentinel lymph node mapping. Ann Surg Oncol 2009;16:2943-52. [Crossref] [PubMed]
- Miyashiro I, Miyoshi N, Hiratsuka M, et al. Detection of sentinel node in gastric cancer surgery by indocyanine green fluorescence imaging: comparison with infrared imaging. Ann Surg Oncol 2008;15:1640-3. [Crossref] [PubMed]
- Noura S, Ohue M, Seki Y, et al. Feasibility of a lateral region sentinel node biopsy of lower rectal cancer guided by indocyanine green using a near-infrared camera system. Ann Surg Oncol 2010;17:144-51. [Crossref] [PubMed]
- Barczyński M, Bränström R, Dionigi G, et al. Sporadic multiple parathyroid gland disease--a consensus report of the European Society of Endocrine Surgeons (ESES). Langenbecks Arch Surg 2015;400:887-905. [Crossref] [PubMed]
- Ferrari CC, Lorenz K, Dionigi G, et al. Surgical strategy for primary hyperparathyreoidism with thyroid hemiagenesis. Langenbecks Arch Surg 2014;399:1077-81. [Crossref] [PubMed]
- Dionigi G, Van Slycke S, Rausei S, et al. Parathyroid function after open thyroidectomy: A prospective randomized study for ligasure precise versus harmonic FOCUS. Head Neck 2013;35:562-7. [Crossref] [PubMed]
- Dionigi G, Boni L, Rausei S, et al. The safety of energy-based devices in open thyroidectomy: a prospective, randomised study comparing the LigaSure™ (LF1212) and the Harmonic® FOCUS. Langenbecks Arch Surg 2012;397:817-23. [Crossref] [PubMed]
- Dionigi G, Bacuzzi A, Bertocchi V, et al. Safe incorporation of new technologies in thyroid surgery. Expert Rev Med Devices 2008;5:747-58. [Crossref] [PubMed]
- Dionigi G, Bacuzzi A, Bertocchi V, et al. Prospectives and surgical usefulness of perioperative parathyroid hormone assay in thyroid surgery. Expert Rev Med Devices 2008;5:699-704. [Crossref] [PubMed]
- Dionigi G, Bacuzzi A, Boni L, et al. Influence of new technologies on thyroid surgery: state of the art. Expert Rev Med Devices 2005;2:547-57. [Crossref] [PubMed]