Diagnostic accuracy of cone-beam breast computed tomography and head-to-head comparison of digital mammography, magnetic resonance imaging and cone-beam breast computed tomography for breast cancer: a systematic review and meta-analysis
Highlight box
Key findings
• This meta-analysis of cone-beam breast computed tomography (CBBCT) showed encouraging diagnostic performance. The CBBCT has greater diagnostic accuracy than digital mammography (DM), although its diagnostic performance is marginally inferior to that of magnetic resonance imaging (MRI).
What is known and what is new?
• CBBCT is a new imaging technique, but it is not yet widely used in clinical diagnosis of breast cancer.
• Our meta-analysis included recent literature, including Chinese literature, to explore the diagnostic efficacy of CBBCT.
What is the implication, and what should change now?
• In the future, more prospective and multi-center studies are needed to further explore the clinical application value of CBBCT.
Introduction
Breast cancer is currently the most prevalent malignant tumor in the world, posing a grave threat to women’s physical and mental health, with an estimated 2.26 million new cases and 68,000 deaths in 2020, accounting for 24.5% of all malignant tumor incidences and 13.6% of female deaths, respectively (1). If these malignancies are diagnosed at an early stage, their death rates may be significantly decreased.
Medical imaging examinations are widely used for tumor screening and diagnosis as they can non-invasively uncover the information contained in a patient’s image. Cone-beam breast computed tomography (CBBCT) is a new breast imaging technology that the U.S. Food and Drug Administration licensed for diagnostic usage in 2015 (2). CBBCT is capable of reconstructing axial, sagittal, coronal, and arbitrary oblique tomographic pictures as well as three-dimensional images of the breast, hence removing tissue overlap on two-dimensional images. CBBCT can quickly scan a single breast without compressing the breast gland. Contrast-enhanced CBBCT (CE-CBBCT) can reveal breast lesions and nearby blood vessels, providing additional imaging data for diagnosis (3-5). However, CBBCT is not currently generally available, and its future use will depend on its diagnostic potential and application value relative to other conventional imaging modalities.
The most widely used clinical imaging modality for early diagnosis of breast cancer is mammography (MG), which is sensitive to calcification, but its limitation is that it is not good at imaging lesions of dense breast tissue (6-8). Breast magnetic resonance imaging (MRI) has the advantages of high soft tissue resolution and the ability to image multiple sequences, parameters, and directions, making it the most sensitive technique for detecting breast lesions, but MRI has limitations due to its long examination time, high price, and obvious noises (7,9-11).
There are limited existing articles assessing the diagnostic efficacy of CBBCT, and different studies have reported variations in the diagnostic accuracy of CBBCT. In a 2019 meta-analysis, Uhlig et al. (12) investigated the diagnostic effectiveness of CBBCT for benign and malignant breast lesions, finding that CE-CBBCT had a pooled sensitivity of 0.899 [95% confidence interval (CI): 0.785–0.956] and a pooled specificity of 0.788 (95% CI: 0.709–0.85). However, recent literature, including Chinese literature, was not included.
In addition, the accuracy of CBBCT in the current meta-analysis was numerically comparable to the accuracy of breast MRI reported in the meta-analysis by Peters et al. (13). Some studies have compared diagnostic accuracy head-to-head between CBBCT and MRI, as well as CBBCT and digital mammography (DM), but no meta-analysis has yet been performed to summarize the findings (14-32).
As a result, the purpose of this study was to conduct a systematic evaluation and analysis of the diagnostic accuracy of CBBCT for breast cancer detection in previous studies. To eliminate the differences between individual studies and explore the value of CBBCT in clinical application, we compared the diagnostic efficacy of CBBCT and DM, and the diagnostic efficacy of CBBCT and MRI in breast lesions. We present this article in accordance with the PRISMA reporting checklist (available at https://gs.amegroups.com/article/view/10.21037/gs-23-153/rc).
Methods
Our research was registered at PROSPERO successfully, and the registration number of this protocol was CRD42022358161 (https://www.crd.york.ac.uk/PROSPERO).
Search strategy
We exhaustively combed the PubMed, Embase, Web of Science, and Chinese Databases through August 2022 in order to locate all relevant material. The keywords were derived from the clusters of terms “breast cancer” and “computed tomography”. The whole search methodology may be found in the Appendix 1. The search for literature was not restricted by period, language, or location. To identify potential relevant research, we manually combed through the reference lists of reputable journals.
Criteria for inclusion and exclusion
Studies were considered for inclusion if all of the following criteria were met: (I) diagnostic clinical trials using CBBCT to assess the malignancy of breast tumors; (II) sufficient data to calculate sensitivity and specificity.
The following items were excluded from consideration: (I) duplicated articles; (II) abstracts; (III) irrelevant titles and abstracts; (IV) data that were unavailable; (V) phantom or simulation studies; (VI) other radiation studies of CBBCT, such as radiotherapy; and (VII) studies involving computer-aided detection (CAD), i.e., machine and deep learning applications in breast cancer diagnostic accuracy.
Using the aforementioned inclusion and exclusion criteria, two researchers independently reviewed the remaining publications’ titles and abstracts to determine their eligibility for the next step. Researchers addressed their disagreements through consensus. The articles selected by mutual decision of the two researchers were acquired in full text for subsequent data extraction analysis.
Quality evaluation
Utilizing the Quality Assessment of Diagnostic Performance Studies-2 (QUADAS-2) instrument, two researchers independently evaluated the included studies’ level of quality. The following criteria were used to evaluate each study: patient selection, index test, reference standard, flow, and timing. The application of these domains was then classified as high, low, or ambiguous based on the risk of bias. Any disagreements were discussed with a third reviewer in order to be resolved.
Data extraction
Data extraction was carried out independently for each included article by two researchers. The consensus among the researchers was used to settle disagreements. The retrieved data included the author, year, study characteristics (country, study design), patient characteristics (number of patients, number of lesions, mean patient age), and technical aspects (equipment, scanner model). The sensitivity and specificity or the matching raw data supplied from each of the included studies were used to calculate the true positive, false negative, false positive, and true negative values.
Statistical analysis
Using random-effect analysis, the combined sensitivity and specificity for CBBCT, MRI, and DM were given as estimates with 95% CIs. The area under the curve (AUC) was computed after the construction of the summary receiver-operating characteristic (SROC) curves. Cochrane Q and I2 statistics were used to evaluate the heterogeneity among the pooled studies. I2 values of 25%, 50%, and 75% were chosen to represent minimum, moderate, and large heterogeneity, respectively. In the case of considerable heterogeneity, meta-regression analysis was used to explore the cause of the heterogeneity. The covariates were (I) mean age of patients included (>50 vs. ≤50 years); (II) number of patients included (>80 vs. ≤80); (III) ethnicity (Asian vs. the rest); (IV) study type (retrospective vs. prospective); (V) type of CBBCT scanner (CBBCT Invented by University of California at San Diego vs. Koning CBBCT); (VI) CBBCT sequence [CE-CBBCT vs. non-contrast CBBCT (NC-CBBCT)]. Publication bias was assessed by Deeks’ funnel plot. Meta-Disc 1.4 was used to analyze whether threshold effects existed in diagnostic meta-analysis. Furthermore, all analyses were carried out using Stata 15.1 (Stata Corporation).
Results
Literature search and study selection
A total of 1,108 publications were found during the original search; after eliminating 393 duplicate research publications, 715 studies remained. Six hundred and eighty-four studies were omitted according to their titles or abstracts. After carefully reviewing the entire texts of the remaining 31 articles, an additional 13 were disqualified for the following reasons: population repeated (n=3); insufficient data could not be retrieved (n=10). The study selection approach is shown in Figure 1 as a PRISMA flow diagram.
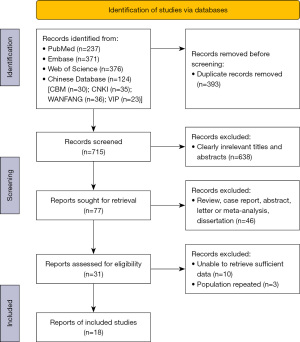
Study description and quality evaluation
The 18 qualifying studies had sample sizes ranging from 30 to 212 and a total of 1,792 patients who had previously undergone CBBCT examination before invasive tests and treatments. They were published before August 2022. Table 1 provides a summary of the study, patient characteristics, and technical elements.
Table 1
Study | Published year | Type of study | Country | No. of patient | No. of lesion | Mean patient age (years) | Equipment | Type of CBBCT | No. of reader [mean years of experience] | Sens. | Specf. | Study intervention |
---|---|---|---|---|---|---|---|---|---|---|---|---|
Aminololama-Shakeri et al. (22) | 2016 | Prospective study | USA | 39 | 39 | 55 | UCSD | CE | 2 [>5] | 16/19 | 19/20 | CBBCT vs. DM |
He et al. (20) | 2016 | Prospective study | China | NC: 92; NC + CE: 120 | NC: 172; NC + CE: 270 | 48 | Koning | NC; CE | 2 [>10] | 97/110 | 279/332 | CBBCT vs. DM vs. US |
Zhao et al. (14) | 2015 | Prospective study | USA | 65 | 85 | 55.6 | Koning | NC | 2 [>7] | 39/45 | 35/40 | CBBCT vs. DM |
Cole et al. (32) | 2015 | – | – | 235 | – | – | Koning | NC | – | 65/144 | 77/91 | CBBCT vs. DM |
Jung et al. (19) | 2017 | Retrospective study | – | 30 | 34 | – | – | – | 4 [7] | 8/8 | 19/26 | CBBCT |
Wienbeck et al. (16) | 2018 | Retrospective study | Germany | 41 | 100 | 57.9 | Koning | NC; CE | 2 [>7] | NC: 29/51; CE: 45/51 | NC: 43/49; CE: 35/49 | CBBCT vs. MRI vs. DM |
Wienbeck et al. (15) | 2017 | Retrospective study | Germany | 65 | 112 | 67.8 | Koning | NC | 2 [18.5] | 70/77 | 12/35 | CBBCT vs. DM |
Chen et al. (21) | 2020 | Prospective study | China | 98 | 100 | 49 | Koning | CE | 1 [15] | 51/74 | 18/26 | CBBCT |
Uhlig et al. (17) | 2019 | Prospective study | Germany | 49 | 100 | 57.9 | Koning | NC + CE; CE | 2 [5] | NC + CE: 49/55; CE: 47/55 | NC + CE: 33/45; CE: 34/45 | CBBCT |
Zhang et al. (25) | 2021 | Retrospective study | China | 46 | 50 | 45 | Koning | CE | 2 [1 senior doctor; 1 junior doctor] | 39/40 | 7/10 | CBBCT vs. MRI |
Zhang et al. (24) | 2021 | Retrospective study | China | 38 | 38 | 44.6 | Koning | CE | 2 [1 senior doctor; 1 junior doctor] | 25/27 | 7/13 | CBBCT vs. MRI |
Liu et al. (28) | 2021 | Retrospective study | China | 97 | 113 | 48.47 | Koning | CE | – | 101/103 | 5/10 | CBBCT vs. DM |
Yang et al. (26) | 2022 | Retrospective study | China | 75 | 83 | 46.8 | Koning | NC | 2 [1 senior doctor; 1 junior doctor] | 35/38 | 42/45 | CBBCT vs. DM |
Zhang et al. (23) | 2021 | Retrospective study | China | 139 | 143 | 48.74 | Koning | CE | 2 [senior doctor] | 120/126 | 10/17 | CBBCT vs. DM |
Liu et al. (29) | 2022 | Retrospective study | China | 78 | 92 | 45.28 | Koning | CE | – | 78/83 | 7/9 | CBBCT vs. MRI |
Zeng et al. (31) | 2020 | Retrospective study | China | 127 | 127 | 47.3 | Koning | – | – | 108/108 | 5/19 | CBBCT vs. US |
Ma et al. (27) | 2021 | Prospective study | China | 198 | 240 | 48 | Koning | CE | – | 135/136 | 73/104 | CBBCT vs. US |
Liu et al. (30) | 2018 | Retrospective study | China | 160 | 165 | 47 | Koning | CE | 2 [1 senior doctor; 1 junior doctor] | 83/87 | 71/78 | CBBCT vs. DM |
CBBCT, cone-beam breast computed tomography; Sens., sensitivity; Specf., specificity; UCSD, CBBCT Invented by University of California at San Diego; CE, contrast-enhanced cone-beam breast computed tomography; DM, digital mammography; NC, non-contrast cone-beam breast computed tomography; Koning, Koning CBBCT; US, ultrasound; MRI, magnetic resonance imaging.
Figure 2 demonstrates our evaluation of these studies regarding the risk of bias according to the QUADAS-2 tool. One of the studies was ranked as having a “high” risk of bias according to the QUADAS-2 recommendations. The included studies’ quality was deemed to be satisfactory.
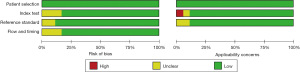
CBBCT diagnostic performance for breast cancer
The study comprised a total of 18 studies with 1,792 patients. No threshold effect was found in this diagnostic meta-analysis (P=0.418). In detecting breast cancer, the combined overall sensitivity and specificity for CBBCT were 0.95 (95% CI: 0.91–0.97) and 0.72 (95% CI: 0.62–0.80) with high heterogeneity (88.36%), respectively (Figure 3). The SROC curve is depicted in Figure 4, and the AUC for CBBCT was 0.92 (95% CI: 0.90–0.94).
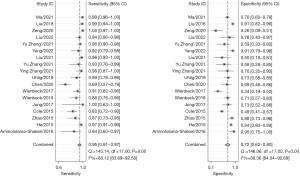
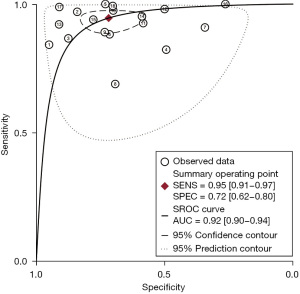
In order to investigate the sources of heterogeneity, meta-regression analysis was conducted, which was not conducted in other studies. We found that ethnicity (P<0.001 for sensitivity), patient count (P<0.001 for sensitivity), study type (P<0.05 for specificity), and type of equipment (P<0.05 for specificity) were potential causes of heterogeneity for CBBCT (Table 2). Figure 5 shows that there was no significant publication bias in the study (P=0.32).
Table 2
Covariate/subgroup | Studies, n | Sensitivity (95% CI) | P value of sensitivity | Specificity (95% CI) | P value of specificity |
---|---|---|---|---|---|
Age | |||||
>50 years | 5 | 0.96 (0.94–0.99) | 0.64 | 0.72 (0.60–0.85) | 0.30 |
≤50 years | 11 | 0.89 (0.80–0.97) | 0.75 (0.58–0.92) | ||
Ethnicity | |||||
Asian | 11 | 0.96 (0.94–0.99) | <0.001 | 0.72 (0.60–0.85) | 0.53 |
The rest | 5 | 0.89 (0.80–0.97) | 0.75 (0.58–0.92) | ||
Study type | |||||
Retrospective | 11 | 0.96 (0.93–0.99) | 0.23 | 0.67 (0.55–0.80) | 0.02 |
Prospective | 6 | 0.91 (0.85–0.98) | 0.81 (0.70–0.93) | ||
Number of patients | |||||
>80 | 8 | 0.92 (0.87–0.97) | <0.001 | 0.76 (0.65–0.88) | 0.76 |
≤80 | 10 | 0.96 (0.92–0.99) | 0.66 (0.52–0.81) | ||
Type of equipment | |||||
USCD | 1 | 0.85 (0.57–1.00) | 0.57 | 0.96 (0.85–1.00) | 0.01 |
Koning | 16 | 0.94 (0.91–0.97) | 0.70 (0.60–0.80) | ||
Type of CBBCT | |||||
NC | 13 | 0.89 (0.79–0.99) | 0.63 | 0.70 (0.49–0.90) | 0.64 |
CE | 4 | 0.95 (0.92–0.98) | 0.72 (0.61–0.84) |
Some articles did not provide information such as age, ethnicity, and type of study, so we did not include these articles in the subgroup analysis. CBBCT, cone-beam breast computed tomography; CI, confidence interval; UCSD, CBBCT Invented by University of California at San Diego; Koning, Koning CBBCT; NC, non-contrast cone-beam breast computed tomography; CE, contrast-enhanced cone-beam breast computed tomography.
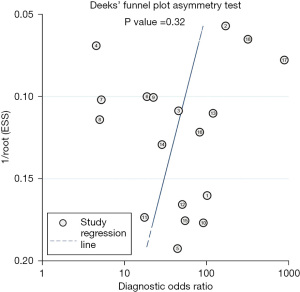
The Fagan nomogram
For CBBCT, the negative post-test probability (the probability of being malignancy when the test is negative) drops to 7% and the positive post-test probability (the probability of being benign when the test is positive) rises to 77% when the pretest probability (prevalence of breast cancer) is assumed to be 50%, which is the medium value of our included studies (Figure 6).
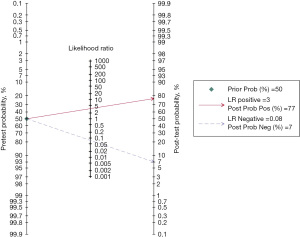
Comparison of CBBCT and DM diagnostic performance for breast cancer
A total of eight studies with 992 subjects were included in the analysis. We aim to head-to-head compare the diagnostic accuracy for breast cancer of CBBCT and DM. The pooled overall sensitivity and specificity for CBBCT in detecting breast cancer were 0.93 (95% CI: 0.89–0.96) and 0.76 (95% CI: 0.60–0.87), while those for DM were 0.78 (95% CI: 0.68–0.86) and 0.75 (95% CI: 0.62–0.84) (Figures 7,8). The SROC curve is depicted in Figure 9, and the AUCs for CBBCT and DM were 0.94 (95% CI: 0.92–0.96) and 0.83 (95% CI: 0.80–0.86), respectively.
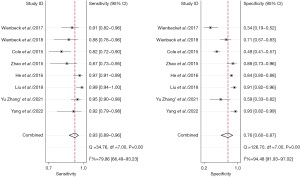
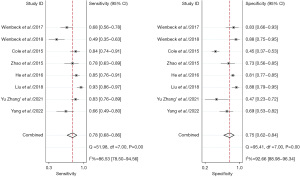
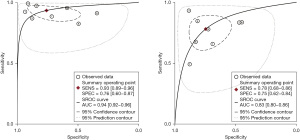
Comparison of CBBCT and MRI diagnostic performance for breast cancer
A total of four studies with 203 patients were included in the analysis. We aim to head-to-head compare the diagnostic accuracy of breast cancer of CBBCT and MRI. The pooled overall sensitivity and specificity for CBBCT in detecting breast cancer were 0.90 (95% CI: 0.79–0.96) and 0.79 (95% CI: 0.65–0.88), while those for MRI were 0.91 (95% CI: 0.79–0.97) and 0.89 (95% CI: 0.72–0.97) (Figures 10,11). Figure 12 shows the SROC curve and the AUC for CBBCT and MRI were 0.88 (95% CI: 0.85–0.91) and 0.96 (95% CI: 0.94–0.97), respectively.
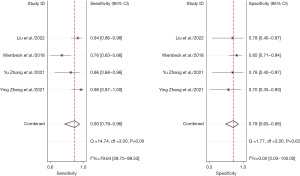
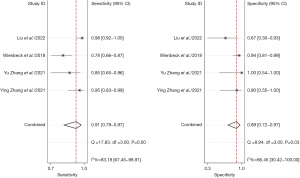
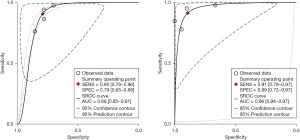
Discussion
A unique breast imaging technique called CBBCT has shown significant diagnostic potential in the detection of breast cancer. Using sensitivity, specificity, and mean AUC of SROC as markers of diagnostic accuracy, we did a systematic review and found 18 published papers on the reliability of CBBCT for the identification of benign and malignant breast lesions. This is the first meta-analysis of direct comparisons of CBBCT and DM, as well as CBBCT and MRI for the diagnosis of benign and malignant breast lesions, to the best of our knowledge.
The pooled overall sensitivity and specificity for CBBCT in identifying breast cancer in this meta-analysis were 0.95 (95% CI: 0.91–0.97) and 0.72 (95% CI: 0.62–0.62), respectively. CBBCT’s SROC curve and AUC were 0.92 (95% CI: 0.90–0.94). When we aggregated overall sensitivity and specificity for CBBCT, we found a lot of variation. Using meta-regression analysis, we investigated the causes of heterogeneity among the studies and their quantitative implications on diagnostic performance. The results show that the ethnicity and the number of patients were significant factors that influenced heterogeneity of sensitivity, study type, and type of CBBCT scanner were possible sources of heterogeneity of specificity. Nevertheless, there may be additional factors, such as differences in the patients, technique, and research design.
In comparison to the results of the meta-analysis presented by Uhlig et al. (12), which had a pooled sensitivity of 78.9%, specificity of 69.7%, and AUC of 0.817, the results of the meta-analysis presented by Komolafe et al. (33) had higher diagnostic efficiency in terms of pooled sensitivity, sensitivity, and mean AUC values (83.7%, 71.3%, and 0.831, respectively). These previous meta-analyses only analyzed literature with small sample sizes and studies in English. In addition, we performed a meta-regression analysis to explore heterogeneity. This meta-analysis yielded the highest diagnostic performance of CBBCT, which may be explained by the recent improvements in imaging technology and the improved level of readers’ skill.
DM was the primary recommendation for breast cancer screening in the National Comprehensive Cancer Network (NCCN) guidelines (34). Head-to-head comparison between CBBCT and DM shows that the SROC curve and the AUC for CBBCT and DM were 0.94 (95% CI: 0.92–0.96) and 0.83 (95% CI: 0.80–0.86) respectively. In our research, it was concluded that the diagnostic efficacy of CBBCT was significantly better than that of DM. In addition, in DM imaging, the density of fibrous glandular tissue can mask tumors and lead to missed diagnosis, and normal glandular tissue may overlap to form tumor-like images. CBBCT reduces the interference of thickness and can detect microlesions equal to 0.2 mm (20).
In addition to the accuracy of the examination, another important evaluation indicator of imaging is radiation dose. Although the radiation dose of CBBCT is slightly higher than that of DM in most studies, the radiation dose of CBBCT for a single scan of a standard breast is 5.8 mGy, which does not exceed the radiation dose standard established by the FDA for DM for screening (total dose not to exceed 6 mGy), and the lifetime attributable risk of cancer induced by this radiation dose is extremely low (35). In the future, improvements anticipate the use of dual-energy approaches for the simultaneous capture of NC-CBBCT and CE-CBBCT in order to decrease radiation dosage (16).
In articles comparing the diagnostic efficacy of CBBCT and MRI, it is usually concluded that there was no statistical difference between the two imaging modalities, and most scholars also consider the diagnostic efficacy of CE-CBBCT to be comparable to that of MRI. However, in this research, head-to-head comparison between CBBCT and MRI shows that the SROC curve and the AUC for CBBCT and MRI were 0.88 (95% CI: 0.85–0.91) and 0.96 (95% CI: 0.94–0.97). In addition, MRI represents an important diagnostic tool to evaluate for axillary lymph node metastasis (36). CBBCT can be used as an imaging option for patients with contraindications to MRI. Other advantages of CBBCT over breast MRI include shorter examination times, less noise, and lower price. Although both imaging modalities require contrast agents with potential adverse effects, it is concerning that the long-term effects of gadolinium-based MRI contrast agents are unknown (37,38).
Calcifications and their morphology then become one of the key factors in the diagnosis of breast cancer. CBBCT has an excellent ability to identify fine, granular, clustered microcalcifications that can help in the diagnosis and management of early breast cancer. However, MRI is not sensitive to breast calcification foci (39). Especially for low-grade ductal carcinoma in situ (DCIS) containing microcalcifications, MRI examination is associated with a 12% false-negative rate due to the lack of enhancement of the lesion (40). Therefore, lesions with suspicious calcification on DM or CBBCT without suspicious enhancement on MRI at the corresponding location need to be treated with caution. The higher soft tissue resolution of MRI combined with the higher spatial resolution of CBBCT and the ability to identify microcalcifications is beneficial for accurate diagnosis of breast cancer, and some studies have combined CBBCT and MRI to achieve better diagnostic performance than individual examinations in several studies (25,29).
CBBCT has not yet become a routine clinical option because there is insufficient evidence-based medical evidence to support its necessity in the diagnostic setting. Future clinical trials of CBBCT in the diagnostic setting of breast cancer are still needed to further evaluate its diagnostic efficacy and define the applicable population. On the other hand, there is still a need to explore the value of CBBCT in breast cancer screening through prospective or retrospective studies due to various factors such as health economics, screening efficacy, and radiation exposure.
It is also necessary to discuss the limitations of our meta-analysis. First, due to the recent introduction of CBBCT as a screening or diagnostic imaging modality, there are no large multicenter prospective or clinical trial studies with standardized acquisition protocols available. This causes variations in the timing of CE-CBBCT acquisition and administration of intravenous contrast material. In addition, no diagnostic criteria and standards for CBBCT have been developed. Most studies have referred to the diagnostic criteria of DM and MRI in breast imaging reporting and data system (BI-RADS) for the diagnosis of CBBCT images. Physicians have less experience in reviewing CBBCT, and the diagnostic agreement between different physicians can be reduced (30). These factors all contribute to an increase in potential bias. Finally, only four studies are included in the head-to-head comparison between CBBCT and MRI, which leads to small sample size. This is because we only included studies that used CBBCT and MRI for the diagnosis of breast cancer within the same patient cohorts to avoid patient selection bias as much as possible.
Conclusions
With promising sensitivity, specificity, and AUC values, our research concludes that the diagnostic performance of CBBCT in our meta-analysis is superior than that in the earlier meta-analysis. There is a tendency toward a greater sensitivity and diagnostic accuracy of CBBCT compared to DM for the diagnosis of breast cancer, according to a meta-analysis of head-to-head comparative studies. In contrast, when MRI and CBBCT were compared side by side, MRI’s diagnostic effectiveness was somewhat superior. We expect that as the underlying imaging physics of this modality are better understood and CAD applications are developed, the diagnostic performance of CBBCT will continue to improve (41-43). More prospective studies comparing the diagnostic accuracy of CBBCT and MRI for breast cancer characterisation and detection are recommended.
Acknowledgments
Funding: None.
Footnote
Reporting Checklist: The authors have completed the PRISMA reporting checklist. Available at https://gs.amegroups.com/article/view/10.21037/gs-23-153/rc
Peer Review File: Available at https://gs.amegroups.com/article/view/10.21037/gs-23-153/prf
Conflicts of Interest: All authors have completed the ICMJE uniform disclosure form (available at https://gs.amegroups.com/article/view/10.21037/gs-23-153/coif). The authors have no conflicts of interest to declare.
Ethical Statement: The authors are accountable for all aspects of the work in ensuring that questions related to the accuracy or integrity of any part of the work are appropriately investigated and resolved.
Open Access Statement: This is an Open Access article distributed in accordance with the Creative Commons Attribution-NonCommercial-NoDerivs 4.0 International License (CC BY-NC-ND 4.0), which permits the non-commercial replication and distribution of the article with the strict proviso that no changes or edits are made and the original work is properly cited (including links to both the formal publication through the relevant DOI and the license). See: https://creativecommons.org/licenses/by-nc-nd/4.0/.
References
- Sung H, Ferlay J, Siegel RL, et al. Global Cancer Statistics 2020: GLOBOCAN Estimates of Incidence and Mortality Worldwide for 36 Cancers in 185 Countries. CA Cancer J Clin 2021;71:209-49. [Crossref] [PubMed]
- O'Connell AM, Karellas A, Vedantham S, et al. Newer Technologies in Breast Cancer Imaging: Dedicated Cone-Beam Breast Computed Tomography. Semin Ultrasound CT MR 2018;39:106-13. [Crossref] [PubMed]
- O'Connell AM, Marini TJ, Kawakyu-O'Connor DT. Cone-Beam Breast Computed Tomography: Time for a New Paradigm in Breast Imaging. J Clin Med 2021;10:5135. [Crossref] [PubMed]
- Zhu Y, O'Connell AM, Ma Y, et al. Dedicated breast CT: state of the art-Part II. Clinical application and future outlook. Eur Radiol 2022;32:2286-300. [Crossref] [PubMed]
- Wei W, Yi XL, Yang J, et al. CT values of contrast-enhanced CBBCT: A useful diagnostic tool for benign and malignant breast lesions. Acta Radiol 2023;64:2379-86. [Crossref] [PubMed]
- Lim ZL, Ho PJ, Khng AJ, et al. Mammography screening is associated with more favourable breast cancer tumour characteristics and better overall survival: case-only analysis of 3739 Asian breast cancer patients. BMC Med 2022;20:239. [Crossref] [PubMed]
- Rahman WT, Helvie MA. Breast cancer screening in average and high-risk women. Best Pract Res Clin Obstet Gynaecol 2022;83:3-14. [Crossref] [PubMed]
- Venkatesan P. New US breast cancer screening recommendations. Lancet Oncol 2023;24:e242. [Crossref] [PubMed]
- Mizzi D, Allely C, Zarb F, et al. Examining the effectiveness of supplementary imaging modalities for breast cancer screening in women with dense breasts: A systematic review and meta-analysis. Eur J Radiol 2022;154:110416. [Crossref] [PubMed]
- Xue F, Jiang J. Dynamic Enhanced Magnetic Resonance Imaging versus Ultrasonic Diffused Optical Tomography in Early Diagnosis of Breast Cancer. J Healthc Eng 2022;2022:4834594. [Crossref] [PubMed]
- Geuzinge HA, Bakker MF, Heijnsdijk EAM, et al. Cost-Effectiveness of Magnetic Resonance Imaging Screening for Women With Extremely Dense Breast Tissue. J Natl Cancer Inst 2021;113:1476-83. [Crossref] [PubMed]
- Uhlig J, Uhlig A, Biggemann L, et al. Diagnostic accuracy of cone-beam breast computed tomography: a systematic review and diagnostic meta-analysis. Eur Radiol 2019;29:1194-202. [Crossref] [PubMed]
- Peters NH, Borel Rinkes IH, Zuithoff NP, et al. Meta-analysis of MR imaging in the diagnosis of breast lesions. Radiology 2008;246:116-24. [Crossref] [PubMed]
- Zhao B, Zhang X, Cai W, et al. Cone beam breast CT with multiplanar and three dimensional visualization in differentiating breast masses compared with mammography. Eur J Radiol 2015;84:48-53. [Crossref] [PubMed]
- Wienbeck S, Uhlig J, Luftner-Nagel S, et al. The role of cone-beam breast-CT for breast cancer detection relative to breast density. Eur Radiol 2017;27:5185-95. [Crossref] [PubMed]
- Wienbeck S, Fischer U, Luftner-Nagel S, et al. Contrast-enhanced cone-beam breast-CT (CBBCT): clinical performance compared to mammography and MRI. Eur Radiol 2018;28:3731-41. [Crossref] [PubMed]
- Uhlig J, Fischer U, Biggemann L, et al. Pre- and post-contrast versus post-contrast cone-beam breast CT: can we reduce radiation exposure while maintaining diagnostic accuracy? Eur Radiol 2019;29:3141-8. [Crossref] [PubMed]
- Liu A, Ma Y, Yin L, et al. Comparison of malignant calcification identification between breast cone-beam computed tomography and digital mammography. Acta Radiol 2023;64:962-70. [Crossref] [PubMed]
- Jung HK, Kuzmiak CM, Kim KW, et al. Potential Use of American College of Radiology BI-RADS Mammography Atlas for Reporting and Assessing Lesions Detected on Dedicated Breast CT Imaging: Preliminary Study. Acad Radiol 2017;24:1395-401. [Crossref] [PubMed]
- He N, Wu YP, Kong Y, et al. The utility of breast cone-beam computed tomography, ultrasound, and digital mammography for detecting malignant breast tumors: A prospective study with 212 patients. Eur J Radiol 2016;85:392-403. [Crossref] [PubMed]
- Chen JT, Zhou CY, He N, et al. Optimal acquisition time to discriminate between breast cancer subtypes with contrast-enhanced cone-beam CT. Diagn Interv Imaging 2020;101:391-9. [Crossref] [PubMed]
- Aminololama-Shakeri S, Abbey CK, Gazi P, et al. Differentiation of ductal carcinoma in-situ from benign micro-calcifications by dedicated breast computed tomography. Eur J Radiol 2016;85:297-303. [Crossref] [PubMed]
- Zhang Y, Liao H, Kang W, et al. Comparison of cone-beam breast CT and mammography in diagnosis of breast cancer. Imaging research and medical applications 2021;5:54-6.
- Zhang Y, Zhang Y, Li J, et al. Diagnostic Efficiency in Breast Lesions Between Cone-Beam Breast CT and MRI: A Comparative Study. Chinese Journal of Medical Imaging 2021;29:309-13.
- Zhang Y, Ma Y. Comparative Study of Cone Beam Breast CT and Breast MRI in Diagnosis of BI-RADS 4 Lesions on X-ray or Ultrasound. Journal of Clinical Radiology 2021;40:1703-8.
- Yang Y, Li J, Li R, et al. Comparison of Detection and Qualitative Analysis of Breast Lesions by Cone-Beam Breast CT and Digital Mammography. Journal of Clinical Radiology 2022;41:44-8.
- Ma A, Kang W, Mo Q, et al. Comparison of color Doppler ultrasound and cone-beam breast computed tomography in patient with breast tumors. J Pract Radiol 2021;37:1977-80.
- Liu Y, Liu H. Diagnostic performance of mammography versus cone-beam breast CT for breast cancer. Imaging research and medical applications 2021;5:49-50+3.
- Liu H, Liu Y. Diagnostic Value of Cone Beam Breast CT with MRI in Breast Cancer. Women's Health Research 2022;5-7:36.
- Liu A, Ma Y, Yin L, et al. Comparison of the diagnostic efficiency in breast malignancy between cone beam breast CT and mammography in dense breast. Chin J Oncol 2018;28:807-12. [PubMed]
- Zeng X, Li H, Liao H, et al. Value of cone-beam breast CT and contrast-enhanced ultrasound in the diagnosis of benign and malignant breast nodules. Oriental Medicated Diet 2020:249.
- Cole E, Campbell A, Vedantham S, et al. Clinical performance of dedicated breast computed tomography in comparison to diagnostic digital mammography. Radiological Society of North America 2015 Scientific Assembly and Annual Meeting; November 29-December 4, 2015; Chicago, IL, USA.
- Komolafe TE, Zhang C, Olagbaju OA, et al. Comparison of Diagnostic Test Accuracy of Cone-Beam Breast Computed Tomography and Digital Breast Tomosynthesis for Breast Cancer: A Systematic Review and Meta-Analysis Approach. Sensors (Basel) 2022;22:3594. [Crossref] [PubMed]
- Kuhl CK. What the Future Holds for the Screening, Diagnosis, and Treatment of Breast Cancer. Radiology 2023;306:e223338. [Crossref] [PubMed]
- Hendrick RE. Radiation doses and cancer risks from breast imaging studies. Radiology 2010;257:246-53. [Crossref] [PubMed]
- Di Paola V, Mazzotta G, Pignatelli V, et al. Beyond N Staging in Breast Cancer: Importance of MRI and Ultrasound-based Imaging. Cancers (Basel) 2022;14:4270. [Crossref] [PubMed]
- Ramalho J, Ramalho M. Gadolinium Deposition and Chronic Toxicity. Magn Reson Imaging Clin N Am 2017;25:765-78. [Crossref] [PubMed]
- Topcuoglu ED, Topcuoglu OM, Semiz Oysu A, et al. Does Gadoterate Meglumine Cause Gadolinium Retention in the Brain of Children? A Case-Control Study. J Magn Reson Imaging 2020;51:1471-7. [Crossref] [PubMed]
- Kim YE, Cha JH, Kim HH, et al. The Accuracy of Mammography, Ultrasound, and Magnetic Resonance Imaging For the Measurement of Invasive Breast Cancer With Extensive Intraductal Components. Clin Breast Cancer 2023;23:45-53. [Crossref] [PubMed]
- Strobel K, Schrading S, Hansen NL, et al. Assessment of BI-RADS category 4 lesions detected with screening mammography and screening US: utility of MR imaging. Radiology 2015;274:343-51. [Crossref] [PubMed]
- Caballo M, Pangallo DR, Sanderink W, et al. Multi-marker quantitative radiomics for mass characterization in dedicated breast CT imaging. Med Phys 2021;48:313-28. [Crossref] [PubMed]
- Tseng HW, Karellas A, Vedantham S. Dedicated cone-beam breast CT: Data acquisition strategies based on projection angle-dependent normalized glandular dose coefficients. Med Phys 2023;50:1406-17. [Crossref] [PubMed]
- Wei W, Zhong W, Kang W, et al. Reference Range of CT Value in NC-CBBCT Based on Female Breast Structure. Curr Med Imaging 2023;19:1523-32. [Crossref] [PubMed]