WW domain binding protein 2 (WBP2) as an oncogene in breast cancer: mechanisms and therapeutic prospects—a narrative review
Introduction
Female breast cancer overtook lung cancer to become the most prevalent cancer worldwide in 2020 (1). Breast cancer is a highly heterogeneous malignancy originating from the epithelial tissue of the breast, which is a major cause of death in women (2). Early and locally advanced breast cancer can be almost cured by surgical resection combined with chemo-radiotherapy, endocrine therapy, and immunotherapy. However, breast cancer’s heterogeneity and drug resistance notably limit the outcome and prognosis of patients, thus leading to the recurrence and metastasis of breast cancer. Patients with advanced metastatic breast cancer can only improve their quality of life with treatment and do not have the opportunity for a complete cure. Compared with the above treatment methods, the advantages of targeted therapy techniques with molecular specificity can be reflected. Targeted therapy has become a hot direction of tumor treatment because of its characteristics of strong pertinence, ready acceptance by patients, and small response. Therefore, it is of great significance to explore new oncogenes and important molecular markers in the molecular biology of breast cancer for the development of effective targeted inhibitors.
The WW domain binding protein 2 (WBP2) gene is a new breast cancer oncogene discovered in recent years. In 1995, WBP2 was pinpointed as a homologous ligand for the Yes-associated protein (YAP) protein in the Hippo pathway (3). In the last 2 decades of research, it has been found to play an important role in promoting breast cancer progression. Protein tyrosine phosphorylation of WBP2 is its major functional switch. Due to the regulation of multiple factors and signal channels, the RNA and protein levels of WBP2 are differentially expressed. In this study, we explore the function of WBP2 by parsing the regulation of WBP2 at the transcriptional, post-transcriptional, and post-translational levels. Phosphorylated WBP2 is a transcriptional coactivator that binds to WW domain-containing proteins, such as YAP (3), estrogen receptor (ER) (4), and progesterone receptor (PR) (5), through 3 C-terminal PPxY (PY) active regions, and activates the latter transcriptional activity to contribute to cancer development. Proteins that do not contain the WW domain can also interact with WBP2.
The binding position and mechanism of WBP2 have been summarized in this paper to help clarify the functional mechanism of WBP2. Moreover, we found that 3 tyrosine activation pathways of WBP2 in breast cancer are closely related to hormone receptor (HR) status. Therefore, we consider that drug resistance in different subtypes of breast cancer is also specific, which may provide new ideas for the treatment and prevention of drug-resistant breast cancer, especially hormonally-mediated breast cancer. In addition, this paper discovered the application prospect of WBP2 in immunotherapy and explored a new way of breast cancer treatment. Finally, we proposed the potential sites of action and combination therapy strategies of WBP2 inhibitors, aiming to provide a reference direction for the development of clinically targeted drugs. We present the following article in accordance with the Narrative Review reporting checklist (available at https://gs.amegroups.com/article/view/10.21037/gs-22-716/rc).
Methods
We searched for literature in the PubMed database. The search and screening processes are shown in Table 1.
Table 1
Items | Specification |
---|---|
Date of search | August 1, 2022 |
Databases and other sources searched | PubMed |
Search formula used | ((WW Domain Binding Protein 2) OR (WBP2)) AND ((breast cancer) OR (breast neoplasms) OR (human cancer)) |
Timeframe | From January 1997 to August 2022 |
Inclusion and exclusion criteria | Retrospective study, preclinical studies, case report and studies not written in English were excluded |
Selection process | Two co-first authors conducted initial screening by title and abstract. The included articles were read in full text. Eventually, all the authors participated in the discussion and received the same opinion |
WBP2 structure and functions
Structure of WBP2 protein
The WBP2 gene, which encodes the WBP2 protein, is located at 17q25. Chromosome 17 is a strongly rearranged chromosome commonly seen in human cancers (6). The WBP2 protein was first detached from the mouse embryo library (3). WBP2 is composed of 261 amino acids with a molecular weight of 26–28 kD (Figure 1). WBP2 contains an N-terminal pleckstrin homologous glucosyltransferase (PH-GRAM) domain and a C-terminal proline-rich (PR) domain. The 2 phosphorylation sites (192 and 231) in the PR domain indicate that it can be activated by tyrosine kinase and is inextricably linked to the WBP2 function. In addition, the 3 PY motifs in the PR domain have been shown to have the function of participating in protein-protein interaction by binding to molecules containing the WW domain, which are called PY1, PY2, and PY3, respectively. The WW domain consists of 38–40 semi-conserved amino acids and was discovered in YAP (7). WBP2 binds more strongly to the WW-PY region than WBP1, the other YAP ligand (8,9).
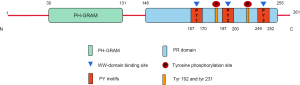
Functions of WBP2
Protein interactions mainly depend on the PY motif at the C-terminal domain of WBP2 for binding to proteins containing the WW domain (10). WBP2 plays a protein-protein interaction through 1 or more PY motifs. For example, the PY2 and PY3 motifs are vital for the WW domain binding interactions of transcriptional coactivator with PDZ-binding motif (TAZ) (11) and YAP (9), respectively. In contrast, the binding of WWC3 is linked to all 3 PY motifs of WBP2 (12). We can mutate different PY motif sites to initially distinguish the relative specificity and binding affinity of different binding substrates.
Coactivation functions belong to a special type of protein interactions, including transcriptional coactivation functions and non-transcriptional coactivation functions. For instance, WBP2 binds to transcription factors like YAP (9), TAZ (11), and β-catenin (13) to perform transcriptional co-activation functions. Further, WBP2 performs non-transcriptional co-activation with other proteins containing the WW domain, such as E6-associated protein (5), WW and C2 domain-containing protein-3 (WWC3) (12), and α-enolase (ENO1) (14). The co-activation activity of WBP2 is unregulated by the absolute regulation of tyrosine phosphorylation at Tyrosine192 (Tyr192) and Tyr231 (10), suggesting that other parts of WBP2 are also crucial for its function.
Recently it has also been reported in the literature that WBP2 can interact with proteins lacking the WW domain, such as large tumor suppressor kinase 2 (LATS2) (15), and paired box 8 (Pax8) (16). Besides, the binding of neural precursor cells which expressed developmentally down-regulated 4 with the PR domain of WBP2 indicated that the regulation of protein stability was probably an alternative mode of transcriptional regulation by WBP2 (17). These pieces of evidence indicate that protein interactions of WBP2 are not only restricted to PY-WW.
WBP2-associated cancers and diseases
Generally, WBP2 is highly expressed in most tumors, especially in breast cancer cells, which demonstrates the broad oncogenic potential of WBP2 in multiple organs. We summarize the biological functions and mechanisms mediated by WBP2 in all tumors in Table 2. WBP2 exerts oncogenic functions in these tumors and is associated with a poor clinical prognosis. Compared with ER or human epidermal growth factor receptor 2 (HER2) positive breast cancer, WBP2 has the highest expression and the worst prognosis in triple-negative breast cancer (TNBC).
Table 2
Tumor | Expression of WBP2 | Interacting proteins/molecules | Correlation with WBP2 | Clinical relevance | Signaling pathway | Biological function | Year | Refs |
---|---|---|---|---|---|---|---|---|
Breast cancer | Upregulated | ERα | Positive | – | ER | Inhibiting apoptosis Promoting doxorubicin resistance | 2018 | (6) |
ERα/c-Src/c-Yes | Positive | – | Wnt | Promoting growth, migration, invasion and tumorigenic | 2011 | (10) | ||
TAZ | Positive | – | Hippo | Promoting growth | 2011 | (11) | ||
ITCH | Negative | Poor prognosis, advanced pTNM stage, larger tumor sizes | Wnt | Promoting growth and invasion | 2016 | (13) | ||
miR-206 | Negative | Poor prognosis | ER | Promoting proliferation and cell cycle Inhibiting apoptosis and tamoxifen sensitivity | 2017 | (18) | ||
miR-613 | Negative | – | EGFR/PI3K/Akt | Promoting growth, proliferation and cell cycle Inhibiting apoptosis | 2018 | (19) | ||
HER2 | Positive | Poor prognosis | EGFR | Promoting growth, proliferation and trastuzumab sensitivity Inhibiting cell cycle | 2019 | (20) | ||
DGCR8 | Negative | Poor prognosis | – | Promoting growth and proliferation | 2021 | (21) | ||
BTRC | Positive | Poor prognosis | NF-κB | Promoting migration and invasion | 2022 | (22) | ||
miR-545-3p/circPRKCI | Negative/positive | Poor prognosis, advanced pTNM stage, larger tumor sizes, positive lymph node metastasis | PI3K/AKT | Promoting proliferation and migration | 2022 | (23) | ||
NSCLC | Upregulated | LATS1 | Negative | Poor prognosis, advanced pTNM stage, positive lymph node metastasis | Hippo | Promoting proliferation and invasion | 2021 | (12) |
Glioma | Upregulated | ENO1, Homer3 | Positive | Advanced pTNM stage | PI3K/Akt | Promoting growth, proliferation, cell cycle, migration and tumorigenic | 2018 | (14) |
Gastric cancer | Upregulated | LATS2 | Negative | Poor prognosis | Hippo | Promoting migration | 2021 | (15) |
cSCC | Upregulated | YAP | Positive | – | Adherens junction | Promoting growth, proliferation Inhibiting differentiation |
2017 | (24) |
HCC | Upregulated | miR-485-5p | Negative | – | Wnt/β-catenin | Promoting proliferation, migration and invasion Inhibiting apoptosis |
2020 | (25) |
WBP2, WW domain binding protein 2; NSCLC, non-small cell lung carcinoma; cSCC, cutaneous squamous cell carcinoma; HCC, hepatocellular carcinoma; ER, estrogen receptor; TAZ, transcriptional coactivator with PDZ-binding motif; ITCH, itchy E3 ubiquitin protein ligase; HER2, human epidermal growth factor receptor 2; DGCR8, DiGeorge Critical Region 8; BTRC, beta-transducin repeat containing E3 ubiquitin protein ligase; ENO1, α-enolase; Homer3, homer protein homolog 3; LATS1/2, large tumor suppressor kinase 1/2; YAP, Yes-associated protein; pTNM, pathologic tumor-node-metastasis; EGFR, epidermal growth factor receptor; PI3K, phosphatidylinositol 3-kinase; Akt/PKB, protein kinase B; NF-κB, nuclear factor kappa B.
Besides human cancers, WBP2 also plays important roles in other diseases. In the nervous system, the absence of WBP2 can cause hearing impairment (26). WBP2 is also associated with neurological diseases like Huntington’s disease and Alzheimer’s disease (27,28). In the endocrine system, WBP2 can be involved in insulin signaling and lipid metabolism (29,30) and may also be involved in thyroid differentiation in conjunction with Pax8 (16). More than that, WBP2 can also be promoted to degradation by itchy E3 ubiquitin-protein ligase (ITCH) to attenuate the proliferation of CD4+ T cells and hence participate in the inflammatory response (31). In addition, WBP2 can activate oocytes, and takes the same position as WW binding protein 2 N-terminal like (WBP2NL) in mouse sperm (32). This finding provides strong evidence for WBP2’s involvement in the development of the reproductive system. Recently, it was revealed that WBP2 was embroiled in apoptosis in monkey kidney cells, suggesting its potential role in the urinary system (33).
Transcriptional regulation of WBP2
Transcriptional regulation by transcription factor upstream transcription factor 1 (USF-1)
USF-1 is the only transcription factor identified so far that regulates WBP2. It contains an USF-specific region (USR) with the T153 residue, which is a functional region of USF-1 involved in transcriptional activation. The T153 residue activates the phosphorylation of USF-1. Phosphorylated USF-1 is further bound to E-box within the core of the WBP2 promoter, thereby increasing WBP2 transcription in breast cancer (30). Although another study demonstrated that USF-1 is a tumor suppressor (34), we believe that USF-1 remains a hazard factor for WBP2-positive breast cancer.
Besides, we believe that USF-1 and WBP2 may act as causative factors in insulin dysregulation-associated cancers because the insulin and phosphatidylinositol 3-kinase (PI3K)/protein kinase B (PKB/Akt) pathways control USF-1 phosphorylation (30). Heightened insulin is correlated with the occurrence and development of several cancers, like pancreatic cancer (35), endometrial cancer (36), and breast cancer (37). In this case, blocking USF-1 and WBP2 loci may be a therapy for cancers associated with impaired insulin metabolism.
Post-transcriptional regulation by NcRNAs
MicroRNAs (miRNAs)
MiRNAs, as a member of non-coding RNAs (ncRNAs), bind to messenger RNA (mRNA) 3' untranslated regions (3' UTRs) to adjust gene expression by either translational inhibition or mRNA degradation. For example, miR-23a (38), miR-206 (18), and miR-613 (19) in breast cancer, and miR-485-5p (25) in hepatocellular carcinoma (HCC) all downregulate WBP2 expression by targeting the 3' UTRs. There is also a reverse regulatory mechanism for the biogenesis process of miRNAs by WBP2. As demonstrated by Tabatabaeian et al., DiGeorge Critical Region 8 (DGCR8) is an essential component of the microprocessor complex, of which the oncogenic properties are inhibited by WBP2, leading to the disruption of downstream mature miRNA formation (21). The mechanism of this process is still unclear. We speculated that WBP2 may regulate the function of DGCR8 through physical binding effects or indirectly by participating in signaling pathways. The biological formation process of miRNAs and the bidirectional regulation of miRNAs and WBP2 are summarized in Figure 2.
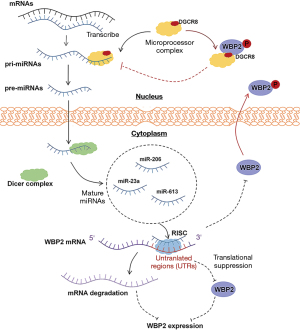
Circular RNAs (circRNAs)
CircRNAs are a class of single-chain closed-loop ncRNAs formed by covalent bonds between the head and the tail. They are expected to be good biomarkers for early diagnosis and prognostic monitoring of cancer (39). CircRNAs that reside in the nucleus can regulate the transcription of their parental genes (40). Most of the circRNAs are spliced and transported into the cytoplasm, acting as sponges for miRNAs and inhibiting their negative regulation of target genes. Some circRNAs are also further transported as vesicles to the extracellular compartment or degraded by ribonuclease (39). CircPRKCI was upregulated in both TNBC tissues and cell lines and localized mainly in the cytoplasm (23). By acting as a sponge for miR-545-3p, circPRKCI has been shown to indirectly upregulate WBP2 and accelerate the proliferation and migration of TNBC in vitro and in vivo (23). This is the only study to verify that circRNAs are involved in the regulation of WBP2 so far.
Long non-coding RNAs (lncRNAs)
LncRNAs are a class of ncRNAs longer than 200 nt, involved in regulating gene expression and multiple cellular processes. Although no lncRNA involvement has been reported in the regulation of WBP2, it has been shown that lncRNAs are involved in regulating WBP2-related miRNAs. For example, lncRNA NEAT1 downregulation of miR-23a-3p expression (41) and lncRNA UCA1 downregulation of miR-613 expression (42) both contribute to paclitaxel resistance in breast cancer; lncRNA UCA1 and FTH1P3 promoted breast cancer proliferation and drug resistance by regulating miR-206 (43,44); lncRNA DSCR8 and LOC554202 acted as molecular sponges of miR-485-5p to promote HCC progression (45,46); and so on. These findings are potential directions for lncRNA’ involvement in WBP2 regulation. Perfecting these studies will benefit to elucidate the mechanistic network of lncRNAs involved in WBP2 regulation.
Post-translational regulation of WBP2
Post-translational modification of proteins is an important step in achieving gene expression, which includes phosphorylation, ubiquitination, and acetylation.
Tyrosine phosphorylation
Tyrosine phosphorylation was identified as the earliest form of post-translational modification of WBP2 (47). WBP2 is tyrosine phosphorylated and is driven to the nucleus by other molecules. High nuclear WBP2 levels have been shown to predict strong tumor aggressiveness and poor prognosis (10). Therefore, it is generally accepted that tyrosine phosphorylation, as a functional switch of WBP2, is important in cancer progression. We mainly summarize 3 pathways of tyrosine phosphorylation activation of WBP2, namely direct activation of epidermal growth factor receptor (EGFR) via EGF, crosstalk of estradiol (E2) and progesterone (P4) with EGFR, and mediation by Wnt ligands (Figure 3).
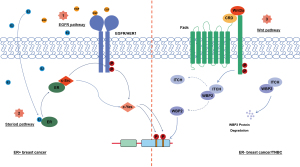
Direct activation of EGFR via EGF
In ER-positive breast cancer, EGFR signaling pathways activate WBP2 tyrosine phosphorylation (10). Both c-Src and c-Yes mediate tyrosine phosphorylation of WBP2 through Tyr192 and Tyr231. Unlike c-Src, c-Yes-induced WBP2 tyrosine phosphorylation was determined by the activation of EGFR (Figure 2). C-Src may function both upstream and downstream of the EGFR during tyrosine phosphorylation of WBP2.
Crosstalk of E2 and P4 with EGFR
EGFR activation was caused by E2 and P4 crosstalk (10). E2 and P4 not only activate the proliferation of breast cancer cells via the nuclear receptor pathway but also have complex signaling communication processes with membrane receptors. Progesterone receptor membrane component 1 (PGRMC1) is overexpressed in both ER-positive and TNBCs (48). It has been shown to interact with the plasma membrane EGFR and increased and stabilized the latter levels (49). E2 and P4 could regulate PGRMC1 expression and then rapidly activate the EGFR pathway downstream of PGRMC1, boosting breast cancer cell proliferation (50). In addition, research has shown that E2 uses the classical steroid signaling pathway to act via ER with c-Src, further stimulating the cleavage and discharge of heparin-binding epidermal growth factor-like growth factor (HB-EGF) (51,52). HB-EGF then activates the EGFR signaling pathway by binding to EGFR via a similar domain to EGF, eventually promoting the phosphorylation of WBP2.
Mediation by Wnt ligands
Different from the EGFR pathway, the Wnt pathway is likely to be the most dominant way for TNBC missing ER expression to mediate tyrosine phosphorylation of WBP2. It is facilitated by Wnt family member 3a disruption of ITCH and WBP2 binding to promote WBP2 protein stability, followed by WBP2 binding to β-catenin to drive transcription mediated by T cell factor (TCF) (13).
Ubiquitination
Protein ubiquitination is another post-translational modification of WBP2 and is an enzymatic reaction. The ubiquitinated proteins are eventually identified and degraded by the proteasome to stabilize the intracellular environment and avert the occurrence of cancer (53). An article reviewed the ubiquitinated substrates of ITCH in various malignancies, including WBP2 (54). ITCH belongs to the HECT-type neuronally expressed developmentally downregulated 4 (NEDD4) family and contains 4 WW domains. It functions as a tumor suppressor in the Wnt signaling pathway of breast cancer, recognizing and degrading WBP2 protein by the PY-WW region (13). The degradation of WBP2 by ITCH could not be completely abolished by proteasome inhibitors, thus suggesting that ITCH may have other modalities mediating the downregulation of WBP2 than by ubiquitination. WWP2, another member of the NEDD4 family, also has a hand in adjusting the ubiquitination of WBP2 through the WW domain (55). Hence, WBP2 may be a good ubiquitination substrate for the NEDD4 family. However, whether other members of this family, such as WW domain-containing E3 ubiquitin-protein ligase 1 and NEDD4, can regulate the ubiquitination of WBP2 protein remains to be investigated.
Signaling pathways of WBP2
The WBP2 protein exerts oncogenic functions by directly participating in Wnt, Hippo, EGFR, and other pathways.
Wnt signaling pathway
The Wnt pathway plays an important role in antitumor response inhibition (56,57). Any abnormalities in this pathway may lead to pathological changes in the body, such as the aberrant initiation of the Wnt pathway in TNBC is imperative for migration and metastasis (58-60).
WBP2 is an important regulator of gene transcription induced by the Wnt pathway; WBP2 regulates nearly 80% of Wnt target genes in TNBC (58). WBP2 ordinarily works as a bridge between the Wnt pathway and other signaling pathways to promote breast cancer progression. Wnt signaling, for example, has been shown to protect WBP2 from ubiquitination degradation of ITCH under Wnt 3a and to drive WBP2 into the nucleus to enhance β-catenin/TCF-mediated transcription, thereby promoting breast cancer progression (13). Indeed, this process also involves the Hippo pathway that mediates the competitive binding of YAP/TAZ to WBP2 and the EGFR signaling pathway that invokes the tyrosine phosphorylation of WBP2 (13). Alternatively, the Wnt and c-Jun N-terminal kinase signaling pathways collaborate to promote TNBC growth via the junction of the WBP2 (58). Wnt cooperates with the Hippo pathway to regulate WBP2 (61-63). Lim et al. predicted that Wnt signaling could downregulate ERα expression to regulate ER signaling when mediated by WBP2 (10). It was advocated that WBP2-mediated breast cancer prefers to rely on the Wnt pathway as opposed to the ER pathway. In conclusion, the Wnt signaling pathway is the backbone of WBP2-mediated breast cancer progression.
As the activation of the Wnt pathway in breast cancer is more complex and frequently implicates the dual effects of both canonical and non-canonical Wnt pathways (64-66), it is uncertain whether the Wnt pathway can be successfully used in breast cancer treatment. Gratifyingly, researchers have mentioned that Wnt pathway-targeted therapy is expected to convert into a true treatment approach (67). Thus, it cannot be ignored that the potential of the Wnt signaling pathway to be a therapeutic target for WBP2-positive breast cancer.
Hippo signaling pathway
The Hippo signaling pathway is committed to suppressing cell proliferation, promoting apoptosis, and regulating the cell cycle (68-70). The core components of Hippo are mainly constitutive of mammalian STE20-like protein kinase 1/2 (MST1/2), LATS1/2, and their adaptor proteins Salvador homologue 1 (SAV1) and MOB kinase activator 1A/B. They inhibit transcriptional coactivator activity of downstream YAP/TAZ through a series of protein cascades, thereby inhibiting in vitro transformation and in vivo tumorigenesis (71,72). Oncogenic factor WBP2 is mutually regulated with the Hippo pathway to promote or restrict cancer progression.
WBP2 inhibits the Hippo pathway in 2 ways
WBP2 as a transcriptional coactivator of YAP/TAZ
The synergy between WBP2 and YAP was initially identified by a functional screen using the complementary DNA (cDNA) expression library (3). WBP2 collaborates with YAP via the PY-WW region to drive YAP transcriptional activity, enhancing the proliferative advantage of YAP in multiple cellular systems, including breast cancer cells (19,68). Moreover, WBP2 cooperates with TAZ in a similar way to YAP to promote TAZ-mediated breast cell transformation (11). Interestingly, p73 was found to competitively bind to YAP with WBP2, because the PY motif contained by p73 is similar to that of WBP2 (73). In contrast to previously identified oncogenic functions, YAP exerts a tumor suppressor effect by binding p73 to promote its transcriptional activity and enhance the induction of apoptosis (74,75). Studying how WBP2 interferes with the tumor suppressive effect of YAP-p73 is important for the exploitation of clinical WBP2-targeted drugs.
WBP2 as an adaptor of LATS1/2
LATS1/2 phosphorylation activation is significant for the proper functioning and maintenance of the Hippo pathway. The WBP2 inhibition of LATS1/2 phosphorylation inhibits the upstream Hippo pathway in a way not directly dependent on YAP.
In gastric cancer, WBP2 is directly bound to LATS2, lacking the WW domain, to constrain the phosphorylation of the Hippo pathway (15). Pull-down assays have demonstrated that WBP2 and LATS2 may function by binding to their respective C-terminal regions (15), providing evidence that WBP2 exerts functional activity with non-WW structural domain dependence. Of course, we cannot rule out the possibility that other molecular signals play an indirect role. In addition to this, it should be considered that WBP2 may have a stabilizing effect on the inactive conformation of LATS2 or that WBP2 acts as a linker protein for LATS2 to non-allosterically inhibit its activity.
In non-small cell lung cancer (NSCLC), WBP2 competes with LATS1 to bind the WW domain of WWC3, inhibits the formation of the LATS1-WWC3 complex, and leads to the downregulation of LATS1 phosphorylation (12). Researchers have summarized the signaling axis of MST1/2-SAV1-WWC1/2/3-LATS1/2-YAP/TAZ (76). As a tumor suppressor in human cancers (77), WWC proteins with a double WW domain should also be important constituents of the Hippo pathway. WWC1 deficiency has been linked to TNBC cell metastasis in multiple publications (78,79) and has been shown to predict poor prognosis (80). It is regrettable that WWC1/2, which is closely associated with breast cancer, has not been reported to interact with WBP2. Future studies can be conducted in this direction to enrich the mechanistic studies of WBP2.
Hippo pathway regulates WBP2 in 2 ways
MST-Dicer signaling axis
MST is required for the expression of Dicer, an enzyme required for pre-miRNA processing. The novel MST-Dicer axis downregulates WBP2 and conspicuously suppresses the WBP2-mediated cell growth and proliferation effects via miR-23a (38). The whole process does not involve the downstream LATS of the MST. Another study demonstrated that LATS could downregulate WBP2 in an MST-independent manner, but was less effective than the MST (81). Furthermore, YAP and TAZ downstream have been shown to actively adjust Dicer expression post-transcriptionally via Let-7 (82), implying that YAP/TAZ is also indirectly engaged in WBP2 regulation by the MST/Dicer axis.
ITCH-mediated proteasomal degradation
ITCH is considered an inhibitor of the Hippo pathway because it mediates LATS1 degradation (83,84). However, the WBP2 degradation induced by ITCH as a tumor suppressor could be reversed by YAP/TAZ overexpression (13). This is because YAP/TAZ and ITCH compete with the PY motif of WBP2. Not only that, the WBP2-ITCH complex can also be inhibited by other signaling pathways such as Wnt and EGFR (13), which further enhances the complexity of the WBP2/Hippo network. More research into the functions of ITCH in different pathways may provide broader evidence support for interactions in the WBP2 and Hippo pathways.
EGFR signaling pathway
The EGFR signaling pathway is a major pathway for cell survival and has been linked to drug resistance (85,86). Positive EGFR expression is frequently associated with poor prognosis of the tumors, including TNBC (87,88).
WBP2 is a true tyrosine phosphorylation target of the EGFR signaling pathway. EGF exhibits a similar tendency to the tyrosine phosphorylation pattern of the WBP2 protein in breast cancer cells (47). Similar to Wnt, EGFR is involved in regulating the protein stability of WBP2 (47). In addition, it has been discovered that the downregulation of WBP2 reduces the expression of the EGFR proteins, as well as pivotal proteins in the EGFR pathway (19). Given the role of WBP2 on the EGFR pathway, further development of combined inhibitors of WBP2 and EGFR pathways is perhaps an effective medicinal strategy for ER-negative breast cancer.
Other pathways
Except for the 3 main signaling pathways studied above, other pathways have also been implicated in the carcinogenic effects of WBP2. For example, the downregulation of WBP2 can reduce the expression of key proteins in the PI3K/AKT pathway and affect the growth and development of breast cancer cells (19). ENO1 and glycolytic virtue are regulated by WBP2 through the ENO1-PI3K/Akt pathway, thus being charged with the occurrence and advancement of glioma (14). WBP2 over-expression induces the adenosine monophosphate-activated protein kinase β1 (AMPKβ1) phosphorylation and activates the AMPK pathway to reduce fat deposition, which provides a new direction for the therapy of non-alcoholic fatty liver disease and insulin resistance (29). Moreover, WBP2 can maintain the mRNA stability of beta-transducin repeat containing E3 ubiquitin-protein ligase in TNBC, resulting in the ubiquitination and degradation of nuclear factor kappa B inhibitor α, and ultimately promoting the invasion and migration induced by WBP2 in TNBC (22).
Taken together, WBP2 is the node that links multiple signaling pathways. WBP2 is activated in response to crosstalk of multiple pathways including ER, EGFR, Wnt and Hippo. Phosphorylated WBP2 entry into the nucleus promotes transcription of oncogenes, thereby promoting cancer progression. The entry of WBP2 into the nucleus is also regulated by phosphorylated WW-containing oxidoreductase (WWOX), which hinders the entry of WBP2 by binding to the PY motif of WBP2, thereby inhibiting WBP2-mediated transcriptional function (5,8) (Figure 4). WBP2 is probably an innovative biomarker and a potential therapeutic target for breast cancer.
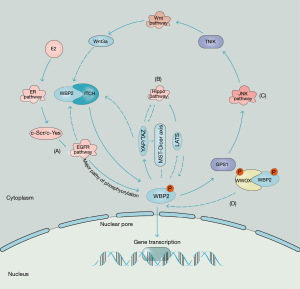
Nuclear WBP2 protein is a potential indicator for the diagnosis and prognosis of breast cancer
WBP2 expression is inconsistent at the protein and mRNA levels in different cancers, as shown by the bioinformatic analysis (27) and the experimental results (13). Especially in hormone-dependent cancers such as breast cancer, high WBP2 protein levels are more common than high WBP2 mRNA expression. Moreover, the high levels of WBP2 protein are more likely present in aggressive breast cancer cell lines and clinical tissues (13). Lim et al. summarized that WBP2 promotes cancer phenotypes in breast cancer cell lines (89). Chen et al. found that WBP2 overexpression caused increased chemotherapy drug resistance in MCF-7 cells (6). These studies indicate that overexpression of WBP2 promotes malignant progression of breast cancer (Figure 5A,5B).
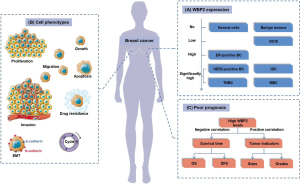
Among the proteins encoded by WBP2, nuclear WBP2 is considered the main functional form of WBP2 after its activation. Lim et al. further analyzed the WBP2 protein levels in the cytoplasm and nucleus by immunohistochemistry and confocal microscopy and found a more significant increase in WBP2 protein in the nucleus compared to normal tissue (13). Moreover, the expression of nuclear WBP2 was negatively correlated with overall survival and disease-free survival, suggesting a worse prognosis for patients with high nuclear WBP2 protein levels (Figure 5C).
In summary, we believe that nuclear WBP2 protein is a vital indicator for the diagnosis and prediction of breast cancer progression. Nonetheless, the detection of the WBP2 protein still requires additional assays for a joint evaluation to improve the reliability of its applications, such as immunofluorescence, immunochemical cytochemical techniques, etc.
WBP2 and breast cancer therapy
Relationships between WBP2 and drug resistance in breast cancer
The high morbidity and mortality rates of breast cancer have long made its treatment a focus of clinicians’ attention. Drug tolerance is now the greatest challenge faced by clinicians. Since WBP2 has an indispensable role in inducing drug resistance in breast cancer, we hope to find new opportunities to develop effective targeted therapeutics from the link of WBP2 to breast cancer drug resistance.
In ER-positive breast cancer
WBP2 is associated with doxorubicin resistance in ER-positive breast cancer. The WBP2 protein was found to be poorly expressed in the ER-positive MCF-7, but highly expressed in the MCF-7 doxorubicin-resistant strains. This differential expression was not appropriate for the ER-negative MDA-MB-231 cell line (6). This indicates that the high expression of WBP2 produced by doxorubicin-resistant cells is related to the positive state of ER. Prolonged stimulation with doxorubicin induces high expression of WBP2 in ER-positive breast cancer cells, and the up-regulation or down-regulation of WBP2 results in altered doxorubicin sensitivity in ER-positive breast cancer (6). This suggests that WBP2 has a potential role in inducing doxorubicin resistance in ER-positive breast cancer. Chen et al. found that WBP2 is directly associated with multidrug resistance gene 1 (MDR1) and demonstrated that WBP2 directly binds to ERα to activate downstream MDR1, which leads to doxorubicin efflux and resistance in ER-positive breast cancer (6). These findings suggest that the WBP2-ERα-MDR1 axis may be a new mechanism of chemotherapy resistance in ER-positive breast cancer.
Upregulation of WBP2 is also closely linked to tamoxifen resistance, the most commonly used chemotherapeutic in ER-positive breast cancer (90). It can compete with estrogen to bind ER, hence restraining the conventional ER transcriptional program (91). However, tamoxifen has been shown to fail to prevent WBP2-induced breast cancer growth and proliferation (10). Ren et al. suggested that ER-positive breast cancer alters tamoxifen sensitivity by adjusting G1/S progression through the miR-206/WBP2 axis (18).
In HER2-positive breast cancer
As we all know, one of the first-line drugs for HER2-positive breast cancer is trastuzumab, but not all patients have been shown to benefit from it (92). It is probably because the response of breast cancer to trastuzumab does individually rely not only on HER2 expression but also on other biomarkers such as WBP2 (20). Kang et al. showed that HER2-positive breast cancer with high WBP2 levels is more effective on trastuzumab (20). It might be due to the combined amplification of HER2 and WBP2 on the q-arm of chromosome 17 and the activation of tyrosine phosphorylation of WBP2 by HER2 in response to the crosstalk of the EGFR signaling pathway. This is not surprising, as it has been found that heterodimerization of EGFR and HER2 occurs in the presence of EGF (47). The above illustrates that WBP2 might be a valid predictor in HER2-positive breast cancer treated with trastuzumab. Moreover, WBP2 co-expression with HER2 in breast cancer patients was connected to a worse prognosis than HER2 expression alone (20). This suggests that WBP2 remains a hazard factor for HER2-positive breast cancer.
In conclusion, WBP2 involves multiple drug resistance mechanisms and is probably a valid target for overcoming multidrug resistance in breast cancer. Although no studies have reported that WBP2 is involved in the chemotherapeutic resistance of TNBC, the high expression of WBP2 in ER-negative MDA-MB-231 may be an important hint (6). If the mechanism of drug resistance in TNBC expressing high WBP2 levels is investigated, it will help to elucidate the role of other hormone-independent factors in inducing drug resistance by WBP2.
Immunotherapy of WBP2 in breast cancer
WBP2 is also being mined as a potential direction for immunotherapy. A study on the proliferative activity of WBP2 promoting CD4+ T cells was published in 2020 (31). CD4+ T cells, which are not fully appreciated in immunotherapy, have great potential for immunotherapy. CD4+ T cells can directly recognize major histocompatibility complex class II antigens that are expressed only by a minority of tumor cells and produce killing effects (93). There is clinical evidence for the involvement of CD4+ T cells in antitumor immunity (94). The experiment demonstrated that reduced stability of WBP2 hindered the entry of CD4+ T cells into the S phase. Moreover, the degradation effect of ITCH on WBP2 in CD4+ cells helped to prevent the excessive proliferation of immune cells. Although the finding that WBP2 is important in regulating immune cells is encouraging, it is still difficult to successfully apply WBP2 to immunotherapy. The specific functions of WBP2 in CD4+ T cell subsets or other immune cells remain to be explored.
Potential synergistic treatments for breast cancer
The rise of immunological biological agents and molecularly targeted agents has expanded the scope of therapeutic options in clinical patients. From 2010 to 2020, the Food and Drug Administration (FDA) approved 26 drug indications for the treatment of clinical metastatic breast cancer, of which only one was for chemotherapy and the remaining 25 were for targeted therapies and immunotherapy (95). The advent of targeted therapies has made it possible to extend the lives of more advanced breast cancer patients.
As WBP2 induces multiple drug resistance in breast cancer, we propose that targeting WBP2 may be a feasible therapeutic strategy, such as WBP2 protein inhibitors targeting the PY motif. Given the limitations of monotherapy, combination therapy is considered to achieve better anticancer outcomes. This is because (I) combination therapies can synergistically target cancer from multiple pathways, addressing the issue of drug unavailability due to tumor heterogeneity and lowering the likelihood of cancer cells developing drug resistance (96). (II) Combination therapies can also mitigate the toxicological response caused by high-dose monotherapy (97). (III) FDA-approved drugs can be used in combination therapies to expedite FDA approval and lower drug discovery and validation costs (95). Therefore, combination therapy is closer to the concept of precision medicine and helps to achieve the goal of individualized therapy.
As shown in Figure 6, we listed 5 directions of combination treatment with WBP2 protein inhibitors: (I) combined targeting of transcription factors that bind to WBP2 to inhibit WBP2 function at the transcriptional levels. (II) Combined use of miRNA agonists and MST/Dicer agonists to promote miRNA biogenesis to inhibit WBP2 activity at the post-transcriptional level. (III) Combined application of E3 ligase activators such as ITCH, which binds tightly to WBP2 and inhibits its phosphorylation activation. (IV) In combination with inhibitors of signaling pathways closely related to WBP2 phosphorylation activation. (V) Appropriate application of novel materials improves the efficiency of drug targeting. Such as new nanocarrier drugs (98), which can be effectively delivered to the tissue site, so the drug dose and frequency of use can be reduced accordingly, thus reducing the toxic reactions of patients.
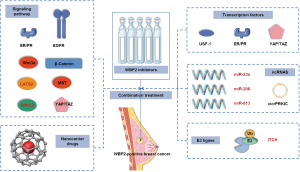
Conclusions
The oncogenic role of WBP2 in human cancers, especially breast cancer, is gradually being revealed. WBP2 functions as an oncogene, regulating breast cancer initiation and development. It was first discovered as a ligand for YAP (3), gradually revealed as a transcriptional coactivator of ER/PR (4,5), then as a new tyrosine kinase substrate in the EGFR pathway (47), and shown to act as a transcriptional activator of YAP/TAZ (11,19), and LATS1/2 (15) phosphorylation inhibitor in the hippo pathway, as a bridge identity linking the Wnt pathway and other pathways (13) and so on. WBP2, under the transcriptional regulation of transcription factors (30) and ncRNAs (18,21,25,38), as well as the post-translational modification of ITCH (13), causes a differential expression of mRNA and protein. Considering the main active form of WBP2, we propose that nuclear WBP2 protein as the standard represents the level of WBP2 and consider nuclear WBP2 as an important indicator for early diagnosis and prognostic monitoring of breast cancer.
From the protein structure of WBP2, the functions of the 2 phosphorylation sites and 3 PY motifs in the C-terminal region are well defined. However, we found that there may be other functional regions at the C-terminus, as WBP2 can interact with proteins without the WW domain. It indicates that the non-PY region at the C-terminus of WBP2 is functioning, but the exact location needs to be explored in depth. In addition, in ER-positive breast cancer, hormones play a very important role in the activation of WBP2 phosphorylation. However, in other hormonal cancers where WBP2 is overexpressed, such as ovarian cancer and prostate cancer, it remains to be explored whether the activation of WBP2 is similarly regulated by hormonal effects.
Targeted therapies are often more precise than traditional chemotherapy in suppressing tumors and destroying tumor mechanisms. Tamoxifen (ER antagonist) and trastuzumab (HER2 antagonist), the first developed and most commonly used in breast cancer, were found to be insensitive to WBP2-overexpressed breast cancers. In this review, we found that, in addition to ER and EGFR pathways, WBP2 activation can also be regulated by Wnt and Hippo pathways. Therefore, we can consider the combination of other pathway inhibitors for the treatment of WBP2-positive breast cancer. The most direct approach is to formulate new targeted drugs against the active structural domain of WBP2, PY, to fundamentally inhibit the activation of WBP2.
Since only about 10% of the human genome can be the target of drugs (99), it remains to be seen whether WBP2 can be used as a drug-targeting molecule. In the future, the combination therapy strategies proposed will help accelerate the development and approval of WBP2-associated drugs. We hope that more and more signaling molecules can be discovered to clarify how WBP2 functions as an oncogene. By testing the gene expression profile of WBP2-positive breast cancer, it will be of great importance to establish a unique molecular profile and customize a personalized therapeutic regimen. This will facilitate more effective treatment and fewer complications for WBP2-positive breast cancer patients. In the future, we may be able to achieve precise treatment of WBP2-positive breast cancer through local biopsy and before and after therapy with WBP2 protein testing.
Acknowledgments
Part of the free illustrations from the website Servier Medical Arts (https://smart.servier.com/).
Funding: This work was supported by the Natural Science Foundation of Sichuan Province (No. 2022NSFSC1584, to Y Wang), Chengdu University of Traditional Chinese Medicine (No. MPRC2021043, to Y Zeng), Chengdu Medical College (No. CYZ19-06, to E He).
Footnote
Reporting Checklist: The authors have completed the Narrative Review reporting checklist. Available at https://gs.amegroups.com/article/view/10.21037/gs-22-716/rc
Conflicts of Interest: All authors have completed the ICMJE uniform disclosure form (available at https://gs.amegroups.com/article/view/10.21037/gs-22-716/coif). All authors report that part of the free illustrations from the website Servier Medical Arts. E He reports grants from Chengdu Medical College (No. CYZ19-06). Y Wang reports grants from Natural Science Foundation of Sichuan Province (No. 2022NSFSC1584). Y Zeng reports grants from Chengdu University of Traditional Chinese Medicine (No. MPRC2021043). The authors have no other conflicts of interest to declare.
Ethical Statement: The authors are accountable for all aspects of the work in ensuring that questions related to the accuracy or integrity of any part of the work are appropriately investigated and resolved.
Open Access Statement: This is an Open Access article distributed in accordance with the Creative Commons Attribution-NonCommercial-NoDerivs 4.0 International License (CC BY-NC-ND 4.0), which permits the non-commercial replication and distribution of the article with the strict proviso that no changes or edits are made and the original work is properly cited (including links to both the formal publication through the relevant DOI and the license). See: https://creativecommons.org/licenses/by-nc-nd/4.0/.
References
- Sung H, Ferlay J, Siegel RL, et al. Global Cancer Statistics 2020: GLOBOCAN Estimates of Incidence and Mortality Worldwide for 36 Cancers in 185 Countries. CA Cancer J Clin 2021;71:209-49. [Crossref] [PubMed]
- Cao W, Chen HD, Yu YW, et al. Changing profiles of cancer burden worldwide and in China: a secondary analysis of the global cancer statistics 2020. Chin Med J (Engl) 2021;134:783-91. [Crossref] [PubMed]
- Chen HI, Sudol M. The WW domain of Yes-associated protein binds a proline-rich ligand that differs from the consensus established for Src homology 3-binding modules. Proc Natl Acad Sci U S A 1995;92:7819-23. [Crossref] [PubMed]
- Buffa L, Saeed AM, Nawaz Z. Molecular mechanism of WW-domain binding protein-2 coactivation function in estrogen receptor signaling. IUBMB Life 2013;65:76-84. [Crossref] [PubMed]
- Dhananjayan SC, Ramamoorthy S, Khan OY, et al. WW domain binding protein-2, an E6-associated protein interacting protein, acts as a coactivator of estrogen and progesterone receptors. Mol Endocrinol 2006;20:2343-54. [Crossref] [PubMed]
- Chen S, Wang H, Li Z, et al. Interaction of WBP2 with ERα increases doxorubicin resistance of breast cancer cells by modulating MDR1 transcription. Br J Cancer 2018;119:182-92. [Crossref] [PubMed]
- Sudol M. Yes-associated protein (YAP65) is a proline-rich phosphoprotein that binds to the SH3 domain of the Yes proto-oncogene product. Oncogene 1994;9:2145-52.
- McDonald CB, Buffa L, Bar-Mag T, et al. Biophysical basis of the binding of WWOX tumor suppressor to WBP1 and WBP2 adaptors. J Mol Biol 2012;422:58-74. [Crossref] [PubMed]
- McDonald CB, McIntosh SK, Mikles DC, et al. Biophysical analysis of binding of WW domains of the YAP2 transcriptional regulator to PPXY motifs within WBP1 and WBP2 adaptors. Biochemistry 2011;50:9616-27. [Crossref] [PubMed]
- Lim SK, Orhant-Prioux M, Toy W, et al. Tyrosine phosphorylation of transcriptional coactivator WW-domain binding protein 2 regulates estrogen receptor α function in breast cancer via the Wnt pathway. FASEB J 2011;25:3004-18. [Crossref] [PubMed]
- Chan SW, Lim CJ, Huang C, et al. WW domain-mediated interaction with Wbp2 is important for the oncogenic property of TAZ. Oncogene 2011;30:600-10. [Crossref] [PubMed]
- Han Q, Rong X, Lin X, et al. WBP2 negatively regulates the Hippo pathway by competitively binding to WWC3 with LATS1 to promote non-small cell lung cancer progression. Cell Death Dis 2021;12:384. [Crossref] [PubMed]
- Lim SK, Lu SY, Kang SA, et al. Wnt Signaling Promotes Breast Cancer by Blocking ITCH-Mediated Degradation of YAP/TAZ Transcriptional Coactivator WBP2. Cancer Res 2016;76:6278-89. [Crossref] [PubMed]
- Chen S, Zhang Y, Wang H, et al. WW domain-binding protein 2 acts as an oncogene by modulating the activity of the glycolytic enzyme ENO1 in glioma. Cell Death Dis 2018;9:347. [Crossref] [PubMed]
- Hum M, Tan HJ, Yang Y, et al. WBP2 promotes gastric cancer cell migration via novel targeting of LATS2 kinase in the Hippo tumor suppressor pathway. FASEB J 2021;35:e21290. [Crossref] [PubMed]
- Nitsch R, Di Palma T, Mascia A, et al. WBP-2, a WW domain binding protein, interacts with the thyroid-specific transcription factor Pax8. Biochem J 2004;377:553-60. [Crossref] [PubMed]
- Jolliffe CN, Harvey KF, Haines BP, et al. Identification of multiple proteins expressed in murine embryos as binding partners for the WW domains of the ubiquitin-protein ligase Nedd4. Biochem J 2000;351:557-65.
- Ren YQ, Wang HJ, Zhang YQ, et al. WBP2 modulates G1/S transition in ER+ breast cancer cells and is a direct target of miR-206. Cancer Chemother Pharmacol 2017;79:1003-11. [Crossref] [PubMed]
- Song H, Wu T, Xie D, et al. WBP2 Downregulation Inhibits Proliferation by Blocking YAP Transcription and the EGFR/PI3K/Akt Signaling Pathway in Triple Negative Breast Cancer. Cell Physiol Biochem 2018;48:1968-82. [Crossref] [PubMed]
- Kang SA, Guan JS, Tan HJ, et al. Elevated WBP2 Expression in HER2-positive Breast Cancers Correlates with Sensitivity to Trastuzumab-based Neoadjuvant Therapy: A Retrospective and Multicentric Study. Clin Cancer Res 2019;25:2588-600. [Crossref] [PubMed]
- Tabatabaeian H, Lim SK, Chu T, et al. WBP2 inhibits microRNA biogenesis via interaction with the microprocessor complex. Life Sci Alliance 2021;4:e202101038. [Crossref] [PubMed]
- Lim YX, Lin H, Chu T, et al. WBP2 promotes BTRC mRNA stability to drive migration and invasion in triple-negative breast cancer via NF-kappaB activation. Mol Oncol 2022;16:422-46. [Crossref] [PubMed]
- Wang X, Song H, Fang L, et al. EIF4A3-mediated circPRKCI expression promotes triple-negative breast cancer progression by regulating WBP2 and PI3K/AKT signaling pathway. Cell Death Discov 2022;8:92. [Crossref] [PubMed]
- Walko G, Woodhouse S, Pisco AO, et al. A genome-wide screen identifies YAP/WBP2 interplay conferring growth advantage on human epidermal stem cells. Nat Commun 2017;8:14744. [Crossref] [PubMed]
- Gao J, Dai C, Yu X, et al. microRNA-485-5p inhibits the progression of hepatocellular carcinoma through blocking the WBP2/Wnt signaling pathway. Cell Signal 2020;66:109466. [Crossref] [PubMed]
- Buniello A, Ingham NJ, Lewis MA, et al. Wbp2 is required for normal glutamatergic synapses in the cochlea and is crucial for hearing. EMBO Mol Med 2016;8:191-207. [Crossref] [PubMed]
- Tabatabaeian H, Rao A, Ramos A, et al. The emerging roles of WBP2 oncogene in human cancers. Oncogene 2020;39:4621-35. [Crossref] [PubMed]
- Fornage M, Debette S, Bis JC, et al. Genome-wide association studies of cerebral white matter lesion burden: the CHARGE consortium. Ann Neurol 2011;69:928-39. [Crossref] [PubMed]
- Zheng Z, Li Y, Fan S, et al. WW domain-binding protein 2 overexpression prevents diet-induced liver steatosis and insulin resistance through AMPKbeta1. Cell Death Dis 2021;12:228. [Crossref] [PubMed]
- Ramos A, Miow QH, Liang X, et al. Phosphorylation of E-box binding USF-1 by PI3K/AKT enhances its transcriptional activation of the WBP2 oncogene in breast cancer cells. FASEB J 2018:fj201801167RR.
- Field NS, Elbulok OA, Dybas JM, et al. Itch attenuates CD4 T-cell proliferation in mice by limiting WBP2 protein stability. Eur J Immunol 2020;50:1468-83. [Crossref] [PubMed]
- Hamilton LE, Suzuki J, Acteau G, et al. WBP2 shares a common location in mouse spermatozoa with WBP2NL/PAWP and like its descendent is a candidate mouse oocyte-activating factor. Biol Reprod 2018;99:1171-83. [Crossref] [PubMed]
- Zhang Y, Jones C, Dickman MB. Identification of differentially expressed genes following treatment of monkey kidney cells with the mycotoxin fumonisin B(1). Food Chem Toxicol 2001;39:45-53. [Crossref] [PubMed]
- Ismail PM, Lu T, Sawadogo M. Loss of USF transcriptional activity in breast cancer cell lines. Oncogene 1999;18:5582-91. [Crossref] [PubMed]
- Bednarz K, Kowalczyk K, Cwynar M, et al. The Role of Glp-1 Receptor Agonists in Insulin Resistance with Concomitant Obesity Treatment in Polycystic Ovary Syndrome. Int J Mol Sci 2022;23:4334. [Crossref] [PubMed]
- Barczynski B, Fraszczak K, Kotarski J. Perspectives of metformin use in endometrial cancer and other gynaecological malignancies. J Drug Target 2022;30:359-67. [Crossref] [PubMed]
- Jafari N, Kolla M, Meshulam T, et al. Adipocyte-derived exosomes may promote breast cancer progression in type 2 diabetes. Sci Signal 2021;14:eabj2807.
- Lim SK, Tabatabaeian H, Lu SY, et al. Hippo/MST blocks breast cancer by downregulating WBP2 oncogene expression via miRNA processor Dicer. Cell Death Dis 2020;11:669. [Crossref] [PubMed]
- Kristensen LS, Jakobsen T, Hager H, et al. The emerging roles of circRNAs in cancer and oncology. Nat Rev Clin Oncol 2022;19:188-206. [Crossref] [PubMed]
- Li W, Liu JQ, Chen M, et al. Circular RNA in cancer development and immune regulation. J Cell Mol Med 2022;26:1785-98. [Crossref] [PubMed]
- Zhu L, Wang F, Fan W, et al. lncRNA NEAT1 promotes the Taxol resistance of breast cancer via sponging the miR-23a-3p-FOXA1 axis. Acta Biochim Biophys Sin (Shanghai) 2021;53:1198-206. [Crossref] [PubMed]
- Liu C, Jiang F, Zhang X, et al. Long Non-Coding RNA UCA1 Modulates Paclitaxel Resistance in Breast Cancer via miR-613/CDK12 Axis. Cancer Manag Res 2020;12:2777-88. [Crossref] [PubMed]
- Li Y, Zeng Q, Qiu J, et al. Long non-coding RNA UCA1 promotes breast cancer by upregulating PTP1B expression via inhibiting miR-206. Cancer Cell Int 2019;19:275. [Crossref] [PubMed]
- Wang R, Zhang T, Yang Z, et al. Long non-coding RNA FTH1P3 activates paclitaxel resistance in breast cancer through miR-206/ABCB1. J Cell Mol Med 2018;22:4068-75. [Crossref] [PubMed]
- Wang Y, Sun L, Wang L, et al. Long non-coding RNA DSCR8 acts as a molecular sponge for miR-485-5p to activate Wnt/β-catenin signal pathway in hepatocellular carcinoma. Cell Death Dis 2018;9:851. [Crossref] [PubMed]
- Yang L, Deng WL, Zhao BG, et al. FOXO3-induced lncRNA LOC554202 contributes to hepatocellular carcinoma progression via the miR-485-5p/BSG axis. Cancer Gene Ther 2022;29:326-40. [Crossref] [PubMed]
- Chen Y, Choong L-Y, Lin Q, et al. Differential Expression of Novel Tyrosine Kinase Substrates during Breast Cancer Development. Molecular & Cellular Proteomics 2007;6:2072-87. [Crossref] [PubMed]
- Ruan X, Zhang Y, Mueck AO, et al. Increased expression of progesterone receptor membrane component 1 is associated with aggressive phenotype and poor prognosis in ER-positive and negative breast cancer. Menopause 2017;24:203-9. [Crossref] [PubMed]
- Ahmed IS, Rohe HJ, Twist KE, et al. Pgrmc1 (progesterone receptor membrane component 1) associates with epidermal growth factor receptor and regulates erlotinib sensitivity. J Biol Chem 2010;285:24775-82. [Crossref] [PubMed]
- Pedroza DA, Subramani R, Tiula K, et al. Crosstalk between progesterone receptor membrane component 1 and estrogen receptor alpha promotes breast cancer cell proliferation. Lab Invest 2021;101:733-44. [Crossref] [PubMed]
- Arpino G, Wiechmann L, Osborne CK, et al. Crosstalk between the estrogen receptor and the HER tyrosine kinase receptor family: molecular mechanism and clinical implications for endocrine therapy resistance. Endocr Rev 2008;29:217-33. [Crossref] [PubMed]
- Razandi M, Pedram A, Park ST, et al. Proximal events in signaling by plasma membrane estrogen receptors. J Biol Chem 2003;278:2701-12. [Crossref] [PubMed]
- Cockram PE, Kist M, Prakash S, et al. Ubiquitination in the regulation of inflammatory cell death and cancer. Cell Death Differ 2021;28:591-605. [Crossref] [PubMed]
- Yin Q, Wyatt CJ, Han T, et al. ITCH as a potential therapeutic target in human cancers. Semin Cancer Biol 2020;67:117-30. [Crossref] [PubMed]
- Jiang H, Thomas SN, Chen Z, et al. Comparative analysis of the catalytic regulation of NEDD4-1 and WWP2 ubiquitin ligases. J Biol Chem 2019;294:17421-36. [Crossref] [PubMed]
- Hong Y, Manoharan I, Suryawanshi A, et al. beta-catenin promotes regulatory T-cell responses in tumors by inducing vitamin A metabolism in dendritic cells. Cancer Res 2015;75:656-65. [Crossref] [PubMed]
- Spranger S, Bao R, Gajewski TF. Melanoma-intrinsic beta-catenin signalling prevents anti-tumour immunity. Nature 2015;523:231-5. [Crossref] [PubMed]
- Li Z, Lim SK, Liang X, et al. The transcriptional coactivator WBP2 primes triple-negative breast cancer cells for responses to Wnt signaling via the JNK/Jun kinase pathway. J Biol Chem 2018;293:20014-28. [Crossref] [PubMed]
- Hiremath IS, Goel A, Warrier S, et al. The multidimensional role of the Wnt/beta-catenin signaling pathway in human malignancies. J Cell Physiol 2022;237:199-238. [Crossref] [PubMed]
- Tewari D, Bawari S, Sharma S, et al. Targeting the crosstalk between canonical Wnt/beta-catenin and inflammatory signaling cascades: A novel strategy for cancer prevention and therapy. Pharmacol Ther 2021;227:107876. [Crossref] [PubMed]
- Imajo M, Miyatake K, Iimura A, et al. A molecular mechanism that links Hippo signalling to the inhibition of Wnt/beta-catenin signalling. EMBO J 2012;31:1109-22. [Crossref] [PubMed]
- Azzolin L, Panciera T, Soligo S, et al. YAP/TAZ incorporation in the beta-catenin destruction complex orchestrates the Wnt response. Cell 2014;158:157-70. [Crossref] [PubMed]
- Quinn HM, Vogel R, Popp O, et al. YAP and beta-Catenin Cooperate to Drive Oncogenesis in Basal Breast Cancer. Cancer Res 2021;81:2116-27. [Crossref] [PubMed]
- Gujral TS, Chan M, Peshkin L, et al. A noncanonical Frizzled2 pathway regulates epithelial-mesenchymal transition and metastasis. Cell 2014;159:844-56. [Crossref] [PubMed]
- Zhang MZ, Ferrigno O, Wang Z, et al. TGIF governs a feed-forward network that empowers Wnt signaling to drive mammary tumorigenesis. Cancer Cell 2015;27:547-60. [Crossref] [PubMed]
- Puvirajesinghe TM, Bertucci F, Jain A, et al. Identification of p62/SQSTM1 as a component of non-canonical Wnt VANGL2-JNK signalling in breast cancer. Nat Commun 2016;7:10318. [Crossref] [PubMed]
- Nusse R, Clevers H. Wnt/beta-Catenin Signaling, Disease, and Emerging Therapeutic Modalities. Cell 2017;169:985-99. [Crossref] [PubMed]
- Zhang X, Milton CC, Poon CL, et al. Wbp2 cooperates with Yorkie to drive tissue growth downstream of the Salvador-Warts-Hippo pathway. Cell Death Differ 2011;18:1346-55. [Crossref] [PubMed]
- Harvey KF, Pfleger CM, Hariharan IK. The Drosophila Mst Ortholog, hippo, Restricts Growth and Cell Proliferation and Promotes Apoptosis. Cell 2003;114:457-67. [Crossref] [PubMed]
- Wu S, Huang J, Dong J, et al. hippo Encodes a Ste-20 Family Protein Kinase that Restricts Cell Proliferation and Promotes Apoptosis in Conjunction with salvador and warts. Cell 2003;114:445-56. [Crossref] [PubMed]
- Maugeri-Sacca M, De Maria R. The Hippo pathway in normal development and cancer. Pharmacol Ther 2018;186:60-72. [Crossref] [PubMed]
- Ortega A, Vera I, Diaz MP, et al. The YAP/TAZ Signaling Pathway in the Tumor Microenvironment and Carcinogenesis: Current Knowledge and Therapeutic Promises. Int J Mol Sci 2021;23:430. [Crossref] [PubMed]
- Levy D, Adamovich Y, Reuven N, et al. The Yes-associated protein 1 stabilizes p73 by preventing Itch-mediated ubiquitination of p73. Cell Death Differ 2007;14:743-51. [Crossref] [PubMed]
- Strano S, Munarriz E, Rossi M, et al. Physical interaction with Yes-associated protein enhances p73 transcriptional activity. J Biol Chem 2001;276:15164-73. [Crossref] [PubMed]
- Basu S, Totty NF, Irwin MS, et al. Akt phosphorylates the Yes-associated protein, YAP, to induce interaction with 14-3-3 and attenuation of p73-mediated apoptosis. Mol Cell 2003;11:11-23. [Crossref] [PubMed]
- Qi S, Zhu Y, Liu X, et al. WWC proteins mediate LATS1/2 activation by Hippo kinases and imply a tumor suppression strategy. Mol Cell 2022;82:1850-64 e7. [Crossref] [PubMed]
- Hoffken V, Hermann A, Pavenstadt H, et al. WWC Proteins: Important Regulators of Hippo Signaling in Cancer. Cancers (Basel) 2021;13:306. [Crossref] [PubMed]
- Knight JF, Sung VYC, Kuzmin E, et al. KIBRA (WWC1) Is a Metastasis Suppressor Gene Affected by Chromosome 5q Loss in Triple-Negative Breast Cancer. Cell Rep 2018;22:3191-205. [Crossref] [PubMed]
- Singh G, Mishra S, Chander H. KIBRA Team Up with Partners to Promote Breast Cancer Metastasis. Pathol Oncol Res 2020;26:627-34. [Crossref] [PubMed]
- Kim SW, Chu J, Do SI, et al. Low KIBRA Expression Is Associated with Poor Prognosis in Patients with Triple-Negative Breast Cancer. Medicina (Kaunas) 2021;57:837. [Crossref] [PubMed]
- Chan EH, Nousiainen M, Chalamalasetty RB, et al. The Ste20-like kinase Mst2 activates the human large tumor suppressor kinase Lats1. Oncogene 2005;24:2076-86. [Crossref] [PubMed]
- Chaulk SG, Lattanzi VJ, Hiemer SE, et al. The Hippo pathway effectors TAZ/YAP regulate dicer expression and microRNA biogenesis through Let-7. J Biol Chem 2014;289:1886-91. [Crossref] [PubMed]
- Salah Z, Itzhaki E, Aqeilan RI. The ubiquitin E3 ligase ITCH enhances breast tumor progression by inhibiting the Hippo tumor suppressor pathway. Oncotarget 2014;5:10886-900. [Crossref] [PubMed]
- Salah Z, Melino G, Aqeilan RI. Negative regulation of the Hippo pathway by E3 ubiquitin ligase ITCH is sufficient to promote tumorigenicity. Cancer Res 2011;71:2010-20. [Crossref] [PubMed]
- Cheng WL, Feng PH, Lee KY, et al. The Role of EREG/EGFR Pathway in Tumor Progression. Int J Mol Sci 2021;22:12828. [Crossref] [PubMed]
- Uribe ML, Marrocco I, Yarden Y. EGFR in Cancer: Signaling Mechanisms, Drugs, and Acquired Resistance. Cancers (Basel) 2021;13:2748. [Crossref] [PubMed]
- Ramlau R, Krawczyk P, Dziadziuszko R, et al. Predictors of EGFR mutation and factors associated with clinical tumor stage at diagnosis: Experience of the INSIGHT study in Poland. Oncol Lett 2017;14:5611-8. [Crossref] [PubMed]
- Rimawi MF, Shetty PB, Weiss HL, et al. Epidermal growth factor receptor expression in breast cancer association with biologic phenotype and clinical outcomes. Cancer 2010;116:1234-42. [Crossref] [PubMed]
- Lim YX, Lin H, Seah SH, et al. Reciprocal Regulation of Hippo and WBP2 Signalling-Implications in Cancer Therapy. Cells 2021;10:3130. [Crossref] [PubMed]
- Ferreira Almeida C, Oliveira A, Joao Ramos M, et al. Estrogen receptor-positive (ER(+)) breast cancer treatment: Are multi-target compounds the next promising approach? Biochem Pharmacol 2020;177:113989. [Crossref] [PubMed]
- Bafna D, Ban F, Rennie PS, et al. Computer-Aided Ligand Discovery for Estrogen Receptor Alpha. Int J Mol Sci 2020;21:4193. [Crossref] [PubMed]
- Nader-Marta G, Martins-Branco D, de Azambuja E. How we treat patients with metastatic HER2-positive breast cancer. ESMO Open 2022;7:100343. [Crossref] [PubMed]
- Ben Khelil M, Godet Y, Abdeljaoued S, et al. Harnessing Antitumor CD4(+) T Cells for Cancer Immunotherapy. Cancers (Basel) 2022;14:260. [Crossref] [PubMed]
- Poncette L, Bluhm J, Blankenstein T. The role of CD4 T cells in rejection of solid tumors. Curr Opin Immunol 2022;74:18-24. [Crossref] [PubMed]
- Arora S, Narayan P, Osgood CL, et al. U.S. FDA Drug Approvals for Breast Cancer: A Decade in Review. Clin Cancer Res 2022;28:1072-86. [Crossref] [PubMed]
- Plana D, Palmer AC, Sorger PK. Independent Drug Action in Combination Therapy: Implications for Precision Oncology. Cancer Discov 2022;12:606-24. [Crossref] [PubMed]
- Dietrich J. Neurotoxicity of Cancer Therapies. Continuum (Minneap Minn) 2020;26:1646-72. [Crossref] [PubMed]
- Tagde P, Najda A, Nagpal K, et al. Nanomedicine-Based Delivery Strategies for Breast Cancer Treatment and Management. Int J Mol Sci 2022;23:2856. [Crossref] [PubMed]
- Hopkins AL, Groom CR. The druggable genome. Nat Rev Drug Discov 2002;1:727-30. [Crossref] [PubMed]