Effect of GATAD1 regulating the SRRM2 gene on recurrence of thyroid tumor and its molecular mechanism
Introduction
Thyroid carcinoma (THCA) mostly originates from thyroid follicular epithelial cells (1,2), and its morbidity is currently showing an annually increasing trend. It has become the main cause of death among head, neck, and endocrine tumors (3). Since the pathological type of tumor is mainly low-grade differentiated thyroid cancer, most patients have a longer survival time after surgery (4,5). However, due to the abundance of thyroid vascular nerves and the complex anatomy and physiological structure of the thyroid, surgery often fails to completely remove cancer tissue, and the probability of recurrence after surgery is high (6,7). The recurrence of TC usually requires a second operation, which will bring increased complications and can easily lead to death (8). Therefore, it is vitally important to accurately predict the recurrence of THCA after surgery.
Transcription factors (TFs) are the convergence point for various signaling pathways in eukaryotic cells. The deregulation of TFs may lead to a range of diseases such as inflammatory disorders, endocrine disorders, cardiovascular disease, and various cancers (9). To date, more than 1,600 TFs have been found, of which 365 TFs have been identified by tumor cell line histochemistry screening and have been studied in depth. The function of TFs is to regulate the expression of downstream genes (10). Other studies have reported that the target gene network of TF microRNA (miRNA) is associated with survival and recurrence of ovarian cancer (11), and that FOXM1 can mediate the proliferation of liver cancer cells by regulating KIF4A (12).
Reports have shown that TFs play a crucial role in the thyroid. For example, HOXA9 has been found to regulate RUNX2 to enhance the ability of papillary thyroid carcinoma (PTC) migration and invasion (13). However, the function of TFs in the recurrence of thyroid cancer has not been reported. In this study, we obtained the highly expressed TFs as well as differentially expressed genes (DEGs) in THCA, based on bioinformatics analysis. Then, we analyzed the clinical effects of TFs and DEGs on the recurrence of THCA patients, and explored the functions of TFs and DEGs in THCA progression. We identified GATAD1 as a novel potential diagnostic biomarker in THCA recurrence patients. The GATAD1-SRRM2 axis was shown to mediate human THCA recurrence progression, which can serve as an underlying therapeutic target for THCA treatment. We present the following article in accordance with the MDAR reporting checklist (available at https://gs.amegroups.com/article/view/10.21037/gs-22-666/rc).
Methods
Bioinformatic analysis of gene expression data
The whole genome expression and survival data of recurring and non-recurring THCA samples were downloaded from The Cancer Genome Atlas (TCGA) database, and the genome-wide differences were analyzed using the R language analysis program (The R Foundation of Statistical Computing, Vienna, Austria). Meanwhile, the list of common TFs and their downstream genes were obtained using the Viper data package of R language. The copy number of TFs and survival map of patients with increased copy numbers of TFs were obtained by cBioPortal database (https://www.cbioportal.org/). The activity bar graph of TFs in thyroid cell lines was obtained by DepMap database (https://depmap.org/portal/). The relationship between GATAD1 and SRRM2 expression levels was analyzed using R software. The study was conducted in accordance with the Declaration of Helsinki (as revised in 2013).
Cell lines and transfection
The HEK293T, FTC133, and TT human THCA cell lines were purchased from the American Typical Culture Collection Center (ATCC; Manassas, VA, USA). The FTC133 cells were cultured in Dulbecco’s modified Eagle medium (DMEM), nutrient mixture F-12 (1:1, by volume) which containing 2% penicillin/streptomycin, 10% fetal bovine serum (FBS), and 1% amphotericin B (14). The TT cells were cultured in F12K medium containing antibiotics, 2 mM glutamine, and 10% FBS (15). All culture reagents were obtained from Gibco (Invitrogen, Life Technologies Inc., Carlsbad, CA, USA). Cells were cultivated at 37 ℃ in an atmosphere with 5% CO2 (16). No mycoplasma contamination was found in the cell lines.
The sequences of negative control short hairpin shRNA (NC shRNA), sh-GATAD1, and sh-SRRM2 were listed as follows: sh-GATAD1, 5'-CGGCTGCTGAAAAGAAAGTCTCCAC-3'; sh-SRRM2, 5'-AAUAACUCGGUUUCGGUGCTT-3'; NC shRNA, 5'-UUCUCCGAACGUGUCACGUTT-3'; The GATAD1 overexpressing recombinant vector pcDNA3.1-GATAD1 and empty plasmid as negative control (NC) were obtained from GenePharma (Shanghai, China). The THCA cells were transiently transfected using Lipofectamine 3000 (Thermo Fisher, Waltham, MA, USA) and polyetherimide for HEK293T cells.
Cell proliferation assay
FTC133, together with TT cells was cultured in 96 well plate. The cells were cultured for 1, 2, 3, and 4 days after attachment. We then added 0.5% 3-(dimethylthiazol-2-yl)-2,5-diphenyltetrazoliumbromide (MTT; Sigma, St. Louis, MO, USA)-containing medium and treated them for 2 hours. The medium was then replaced with 1 mg/mL dimethyl sulfoxide (DMSO; Sigma, USA). The optical density (OD) value was measured at 490 nm.
Colony formation assay
We cultured lentivirus-infected THCA cells in 6-well plates for 7 days. The cells were then stained with 0.5% (w/v) crystal violet, imaged by scanner, and quantified by ImageJ software (National Institutes of Health, Bethesda, MD, USA).
Western blot
The whole cell lysates were centrifuged for 10 minutes at 4 ℃, after which the bicinchoninic acid (BCA; Pierce, Rockford, IL, USA) method was used to test protein concentrations. Protein was separated using sodium dodecyl sulfate polyacrylamide gel (SDS-PAGE) and transferred to a polyvinylidene fluoride (PVDF) membrane (Bio-Rad, Shanghai, China). The membrane was sealed with 5% skimmed milk powder, and dyed overnight with the primary antibody at 4 ℃. Then, we probed the membranes with a peroxidase conjugated secondary antibody (1:10,000 dilution). We also used enhanced chemiluminescence (ECL) reagent (Pierce, USA) to test the antigen-antibody complexes. Primary antibodies against SRRM2, GATAD1, and GAPDH were obtained from Sigma-Aldrich (Milan, Italy).
Real-time quantitative polymerase chain reaction (RT-qPCR)
Total RNA was extracted from different cell lines using TRIzol reagent (Invitrogen, USA), and 1 µg extracted RNA was reverse transcribed to complementary DNA (cDNA), using a kit from Promega (Madison, WI, USA), according to the manufacturer’s instructions. RT-qPCR was performed by Fast Start universal SYBR Green Master (Roche, Indianapolis, IN, USA). All q-PCR was carried out in triplicate. 2−ΔΔCT was used to evaluate the expression levels in comparison to the control. Table 1 shows the sequences of RT-qPCR primer.
Table 1
Gene | Forward (5'-3') | Reverse (3'-5') |
---|---|---|
GATAD1 | TCTCTAGCCCCAGAGACCAA | AATGGTTGGCAACTGATTCC |
GAPDH | CTTCATTGACCTCAACTACATGG | CTCGCTCCTGGAAGATGGTGAT |
SRRM2 | GAAGAAAGGCCTGCTGTGTC | CCAAAGCTGTTCTCCCTGAG |
Luciferase assays
We transfected pRL-TK and pGL4.2 basic Luc plasmids into HEK293T cells. The GATAD1 expression plasmid or empty body were co transfected for 2 days, and the gene activity was analyzed. The experiment was repeated 3 times.
Cell cycle analysis
The lentivirus-infected THCA cells were harvested, washed in phosphate-buffered saline (PBS), and then fixed it for 2 hours at −20 ℃ with cold 70% ethanol. The cells were centrifuged for 3 minutes, washed in PBS 3 times, suspended in 0.5 mL PBS, and added to 100 µg/mL RNase A (Sigma Chemicals, St. Louis, MO, USA) and 50 µg/mL propidium iodide (PI; Sigma Chemicals) for 30 minutes at 4 ℃ in the dark. Flow cytometry was used to analyze the contents of DNA.
Statistical analysis
The software SPSS 23.0 (IBM Corp., Armonk, NY, USA) was used for statistical analysis. The final data were presented as the mean ± SD of 3 separate studies. To compare 2 or 3 groups, the analysis of variance (ANOVA) or Student’s t-test were utilized, accordingly. A P value less than 0.05 was regarded statistically significant.
Results
Increased GATAD1 activity in THCA
We obtained 365 common TFs from the original gene expression data of recurrent (47 cases) and non-recurrent (65 cases) THCA, using the R language analysis program. The genome-wide differences were analyzed, which showed that GATAD1 was significantly up regulated (Figure 1A). The activity of GATAD1 was remarkably increased in patients with THCA recurrence (Figure 1B), and its expression level was also remarkably increased (Figure 1C). The level of GATAD1 messenger RNA (mRNA) was positively correlated with the number of copies of GATAD1 (Figure 1D). The impact of GATAD1 on THCA patients was revealed by Kaplan-Meier analysis. In specimens of recurrent (47 cases) and non-recurrent (65 cases) THCA, those with GATAD1 gene magnification had a shorter survival time (Figure 1E). These findings indicated that the amplification of GATAD1 copy number leads to the high expression of GATAD1, indicating a high recurrence rate of THCA.
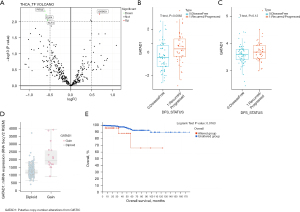
GATAD1 promotes THCA cell proliferation
We conducted MTT assays and cell flow cytometry experiments to investigate the impact of GATAD1 on the proliferation of THCA cells. Firstly, the activity bar graph of GATAD1 in THCA cell lines was obtained through the DepMap database (Figure 2A), showing that it inhibited proliferation of FTC133 (Figure 2B) and TT (P<0.05) (Figure 2C), and arrested FTC133 cells in the G1 phase (Figure 2D,2E).
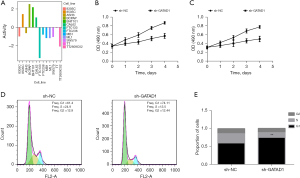
SRRM2 is a key downstream gene of GATAD1
To screen the key downstream genes of GATAD1, 112 THCA patients (47 cases of recurrence and 65 cases of non-recurrence) were divided into different groups. We obtained 4,124 up-regulated genes according to the activity of GATAD1. A total of 926 up-regulated genes were found according to GATAD1 mRNA expression levels. The overall survival analysis of the activity of GATAD1 yielded 1,601 differential genes. Moreover, there were a total of 26 genes satisfying 3 conditions at the same time (Figure 3A). The binding motif prediction of GATAD1 was obtained by the previous reports (Figure 3B). Through the binding motif screening of GATAD1, we discovered a total of 16 downstream genes with binding motif. Among them, SRRM2 was the most highly expressed, and the difference was significantly up-regulated (Figure 3C). According to the expression level of SRRM2 in 112 THCA patients (47 cases of recurrence and 65 cases of non-recurrence), the specimens were divided into low and high expression groups, and it was found that specimens with high SRRM2 expression had poor survival (P=0.019) (Figure 3D,3E).
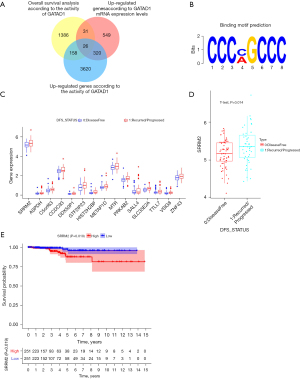
SRRM2 promotes THCA cell proliferation
We conducted MTT assays, cell clone formation assays, and cell flow cytometry experiments to evaluate the impact of SRRM2 on the proliferation of THCA cells. The FTC133 and TT cell lines were selected for experiments. It was revealed that SRRM2 inhibited the proliferation of FTC133 (Figure 4A) and TT (P<0.05) (Figure 4B), and arrested FTC133 cells in the G1 phase (Figure 4C,4D).
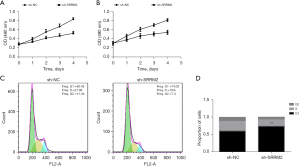
GATAD1 positively regulates SRRM2 expression
To determine whether GATAD1 regulates the expression of SRRM2 in THCA, the findings of bioinformatics analysis revealed that the expression of GATAD1 was positively associated with SRRM2 (Figure 5A). Furthermore, we transfected TT cell lines with the FOXM1 overexpression structure, which promoted SRRM2 protein as well as mRNA levels (Figure 5B-5D). On the contrary, shRNA-mediated knockdown of GATAD1 reversed the result (Figure 5E-5G), indicating that GATAD1 regulated SRRM2 expression.
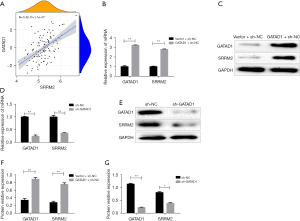
GATAD1 binds to the SRRM2 promoter
The promoter of the SRRM2 gene has 3 conserved CCCNNCCC regions (BS1, −840 to −832; BS2, −758 to −750; BS3, −117 to 109), and GATAD1 can bind to the CCCNNCCC sequence of chromatin. It was speculated that GATAD1 promoted the transcription of the SRRM2 gene by recognizing the conserved region of the SRRM2 gene. To confirm this hypothesis, a different luciferase reporter was transfected into 293T cells with high level GATAD1, and the findings revealed that the full-length promoter construction showed increased luciferase activity (SRRM2 Pro Δ BS1 + BS2, BS3), indicating that GATAD1 directly activated SRRM2 gene transcription. Several extensions of the TSS core promoter, including BS1, BS2, and BS3, can enhance transcription (Figure 6). All the results showed that GATAD1 enhanced SRRM2 transcription by binding BS1, BS2, and BS3.
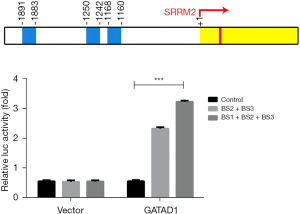
GATAD1 stimulates THCA cell proliferation in a SRRM2-dependent manner
According to the above findings, we inferred that SRRM2 exerts the effect of GATAD1 in the progression of THCA. To verify this hypothesis, SRRM2 was knocked down in GATAD1-overexpressing TT cells. The upregulation of SRRM2 induced with GATAD1 overexpression was abolished at the protein as well as mRNA level (Figure 7A-7C). The cell proliferation experiments also showed similar results. These results indicated that the GATAD1-SRRM2 axis promoted THCA progression and that interfering with the expression of SRRM2 can block GATAD1-mediated THCA cell proliferation (Figure 7D).
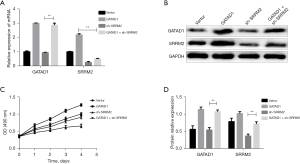
Discussion
Research shows that TFs play a crucial role in cell growth (17). Mounting evidence shows that these proteins are closely related to the genetic level that controls the development of cancer (11), and may provide selective targets for new drug interventions (12,18). Recent drug reports have indicated that TFs are easily inhibited by drugs (9). Many currently used anticancer drugs exert their effects by regulating the activity of important TFs (19). In this study, we found that the activity and expression of GATAD1 were remarkably increased in patients with recurrent THCA. The mRNA of GATAD1 was positively correlated with the number of copies of GATAD1. The survival time of patients with recurrent THCA caused by GATAD1 gene amplification was shorter. The data showed that the amplification of GATAD1 copy number leads to the high expression of GATAD1, and the recurrence rate of THCA is high. Previous studies have shown that GATAD1 was significantly up-regulated in hepatocellular carcinoma (HCC) (20) and glioma (21) through gene amplification. A study revealed that GATAD1 regulated cell cycle via PRL3 (20). We also found that GATAD1 was overexpressed in recurrent THCA cases, which further suggested that GATAD1 is crucial in the THCA recurrence process. Knockdown of GATAD1 reduced the number of THCA cell clones and inhibited the THCA cell proliferation, and arrested THCA cells in the G1 phase.
We found that SRRM2 could bind to the binding motif of GATDA1, and the expression level of SRRM2 was remarkably up-regulated. SRRM2 is a susceptible gene of THCA (22,23), and its mutation can accelerate the formation of THCA (24), but its specific mechanism is still unclear. We found that the recurrent THCA patients with high SRRM2 expression showed worse survival, based on bioinformatics analysis. Knockdown of SRRM2 also reduced the number of THCA cell clones and inhibited the THCA cell proliferation, and arrested THCA cells in the G1 phase. According to the findings, we speculated that SRRM2 is also important in the recurrence of THCA.
Bioinformatics findings revealed that the expression of GATAD1 was positively associated with SRRM2. In addition, FOXM1 overexpression was used to construct transfected TT cell lines, which increased SRRM2 protein and mRNA levels. In contrast, shRNA-mediated GATAD1 knockout reversed these effects, indicating that GATAD1 regulates SRRM2 expression (21). In this study, we discovered 3 conservative CCCNNCCC sequences located in the upstream region of the SRRM2 promoter. The results of the luciferase reporter indicated that GATAD1 directly activated SRRM2 gene transcription. Based on the above observations, we inferred that SRRM2 exerted the effect of GATAD1 in the progression of THCA. To verify this hypothesis, SRRM2 was knocked down in GATAD1-overexpressing THCA cells. The upregulation of SRRM2 induced by GATAD1 overexpression was abolished at the protein and mRNA levels. Cell cloning and cell proliferation experiments also showed similar results. These results indicated that the GATAD1-SRRM2 axis promoted THCA progression and interfered with the expression of SRRM2, resulting in the blockade GATAD1-mediated THCA cell proliferation.
In summary, based on bioinformatics analysis, we screened out the transcription factor GATAD1 which is highly expressed in THCA and its key downstream gene SRRM2. To investigate further, we performed a Kaplan-Meier analysis revealed the effect of GATAD1 on the survival of THCA patients and through cell proliferation and cell cycle experiments, it was proved that GATAD1 promoted the proliferation of cancer cells and caused the recurrence of THCA by up-regulating its downstream gene SRRM2, which confirmed that GATAD1 could be used as a biomarker for the diagnosis of patients with THCA recurrence and an independent predictor of patient survival. This provides a strong theoretical basis for the diagnosis and treatment of recurrent THCA patients. In addition, it has been shown in the past that transcription factors are susceptible to drug inhibition, which means that GATAD1 may become a drug therapeutic target for THCA, improving the current situation of high recurrence rate of THCA and extending the survival time of THCA patients.
Conclusions
We found that GATAD1 was highly expressed in relapsing THCA tissues, and experimentally demonstrated that the GATAD1-SRRM2 axis mediated THCA recurrence progression through promoting cell proliferation, revealing the possibility of GATAD1 as a biomarker for the diagnosis of THCA recurrence and a therapeutic target for THCA.
Acknowledgments
Funding: The study was supported by Scientific Research Project of Heilongjiang Municipal Commission of Health and Family Planning (No. 2020-328).
Footnote
Reporting Checklist: The authors have completed the MDAR reporting checklist. Available at https://gs.amegroups.com/article/view/10.21037/gs-22-666/rc
Data Sharing Statement: Available at https://gs.amegroups.com/article/view/10.21037/gs-22-666/dss
Conflicts of Interest: All authors have completed the ICMJE uniform disclosure form (available at https://gs.amegroups.com/article/view/10.21037/gs-22-666/coif). The authors have no conflicts of interest to declare.
Ethical Statement: The authors are accountable for all aspects of the work in ensuring that questions related to the accuracy or integrity of any part of the work are appropriately investigated and resolved. The study was conducted in accordance with the Declaration of Helsinki (as revised in 2013).
Open Access Statement: This is an Open Access article distributed in accordance with the Creative Commons Attribution-NonCommercial-NoDerivs 4.0 International License (CC BY-NC-ND 4.0), which permits the non-commercial replication and distribution of the article with the strict proviso that no changes or edits are made and the original work is properly cited (including links to both the formal publication through the relevant DOI and the license). See: https://creativecommons.org/licenses/by-nc-nd/4.0/.
References
- Haroon Al Rasheed MR, Xu B. Molecular Alterations in Thyroid Carcinoma. Surg Pathol Clin 2019;12:921-30. [Crossref] [PubMed]
- Mahmoudian-Sani MR, Jalali A, Jamshidi M, et al. Long Non-Coding RNAs in Thyroid Cancer: Implications for Pathogenesis, Diagnosis, and Therapy. Oncol Res Treat 2019;42:136-42. [Crossref] [PubMed]
- Jeong YM, Cho H, Kim TM, et al. CD73 Overexpression Promotes Progression and Recurrence of Papillary Thyroid Carcinoma. Cancers (Basel) 2020;12:3042. [Crossref] [PubMed]
- Hu D, Huang Y, Zeng W, et al. Is a simplified TNM staging system more clinically relevant than the American Joint Committee on Cancer system for the follicular variant of papillary thyroid cancer? Ann Transl Med 2020;8:463. [Crossref] [PubMed]
- Laschinsky C, Herrmann K, Fendler W, et al. Oncological theranostics in nuclear medicine. Radiologie (Heidelb) 2022;62:875-84. [Crossref] [PubMed]
- Giordano D, Frasoldati A, Kasperbauer JL, et al. Lateral neck recurrence from papillary thyroid carcinoma: Predictive factors and prognostic significance. Laryngoscope 2015;125:2226-31. [Crossref] [PubMed]
- Sondermann A, Andreghetto FM, Moulatlet AC, et al. MiR-9 and miR-21 as prognostic biomarkers for recurrence in papillary thyroid cancer. Clin Exp Metastasis 2015;32:521-30. [Crossref] [PubMed]
- Wang M, Zou X, Li Z, et al. Recurrence of papillary thyroid carcinoma from the residual pyramidal lobe: a case report and literature review. Medicine (Baltimore) 2019;98:e15210. [Crossref] [PubMed]
- Papavassiliou KA, Papavassiliou AG. Transcription Factor Drug Targets. J Cell Biochem 2016;117:2693-6. [Crossref] [PubMed]
- Lambert SA, Jolma A, Campitelli LF, et al. The Human Transcription Factors. Cell 2018;172:650-65. [Crossref] [PubMed]
- Bushweller JH. Targeting transcription factors in cancer - from undruggable to reality. Nat Rev Cancer 2019;19:611-24. [Crossref] [PubMed]
- Hu G, Yan Z, Zhang C, et al. FOXM1 promotes hepatocellular carcinoma progression by regulating KIF4A expression. J Exp Clin Cancer Res 2019;38:188. [Crossref] [PubMed]
- Jin Y, Kim HK, Lee J, et al. Transcription Factor HOXA9 is Linked to the Calcification and Invasion of Papillary Thyroid Carcinoma. Sci Rep 2019;9:6773. [Crossref] [PubMed]
- Melnik Daniela, Krüger Marcus, Schulz Herbert, et al. The CellBox-2 Mission to the International Space Station: Thyroid Cancer Cells in Space. Int J Mol Sci 2021;22:8777. [Crossref] [PubMed]
- Giardino E, Catalano R, Barbieri AM, et al. Cofilin is a mediator of RET-promoted medullary thyroid carcinoma cell migration, invasion and proliferation. Mol Cell Endocrinol 2019;495:110519. [Crossref] [PubMed]
- Gu JJ, Hernandez-Ilizaliturri FJ, Mavis C, et al. MLN2238, a proteasome inhibitor, induces caspase-dependent cell death, cell cycle arrest, and potentiates the cytotoxic activity of chemotherapy agents in rituximab-chemotherapy-sensitive or rituximab-chemotherapy-resistant B-cell lymphoma preclinical models. Anticancer Drugs 2013;24:1030-8. [Crossref] [PubMed]
- Papavassiliou AG. Transcription-factor-modulating agents: precision and selectivity in drug design. Mol Med Today 1998;4:358-66. [Crossref] [PubMed]
- Karamouzis MV, Gorgoulis VG, Papavassiliou AG. Transcription factors and neoplasia: vistas in novel drug design. Clin Cancer Res 2002;8:949-61.
- Karamouzis MV, Papavassiliou AG. Transcription factor networks as targets for therapeutic intervention of cancer: the breast cancer paradigm. Mol Med 2011;17:1133-6. [Crossref] [PubMed]
- Sun W, Zhang Y, Wong KC, et al. Increased expression of GATA zinc finger domain containing 1 through gene amplification promotes liver cancer by directly inducing phosphatase of regenerating liver 3. Hepatology 2018;67:2302-19. [Crossref] [PubMed]
- Zhang S, Gao M, Yu L. GATAD1 gene amplification promotes glioma malignancy by directly regulating CCND1 transcription. Cancer Med 2019;8:5242-53. [Crossref] [PubMed]
- Hińcza K, Kowalik A, Kowalska A. Current Knowledge of Germline Genetic Risk Factors for the Development of Non-Medullary Thyroid Cancer. Genes (Basel) 2019;10:482. [Crossref] [PubMed]
- Cirello V. Familial non-medullary thyroid carcinoma: clinico-pathological features, current knowledge and novelty regarding genetic risk factors. Minerva Endocrinol (Torino) 2021;46:5-20. [Crossref] [PubMed]
- Tomsic J, He H, Akagi K, et al. A germline mutation in SRRM2, a splicing factor gene, is implicated in papillary thyroid carcinoma predisposition. Sci Rep 2015;5:10566. [Crossref] [PubMed]
(English Language Editor: J. Jones)